Energy Units
What is a Joule (J)?
In the universe of energy, the Joule (J) takes center stage. It’s the standard unit of energy in the International System of Units (SI). Named after the physicist James Prescott Joule, it measures the energy used to apply a force of one Newton over a distance of one meter.
Think about this – if you’ve ever lifted an apple one meter off the ground, you’ve exerted about 1 Joule of energy. A Joule might seem small, but it’s the building block for all energy measurements.
Larger Units of Energy
For larger amounts of energy, we use kilojoules (kJ), megajoules (MJ), and even gigajoules (GJ). To give you some perspective, the average lightning bolt carries about 1 gigajoule of energy – talk about a shocking fact!
What’s a Calorie?
You’ve probably seen ‘Calories’ listed on the back of a food package. It’s another way we measure energy. When we say something has 100 Calories (note the capital C), we mean it provides 100 kilocalories of energy – or about 418,000 Joules. That’s enough energy to power your phone for a whole day!
Specialized Energy Units
In certain fields, we use very specialized units. One such unit is the electronvolt (eV) which is often used in the realm of atomic physics. It represents the energy an electron gains when it moves through an electric potential difference of one volt. It’s equal to approximately 0.00000000000000000016 joules. Tiny, right?
Energy Units in Real Life
From charging your phone to filling up your car, you’re using energy. The energy your phone battery holds is measured in milliamp-hours (mAh), which can be converted to Joules. The petrol or diesel you put in your car has an energy value too, typically measured in megajoules or gigajoules.
Energy Transfer
What is Energy Transfer?
Energy Transfer is like a game of “pass the parcel.” Just like the parcel is passed around in a circle, energy can be moved from one place to another or from one form to another.
Imagine a hot cup of tea – when you hold the cup, you can feel the heat. This is energy transferring from the hot tea to your hands. In this case, the transfer method is conduction.
Types of Energy Transfer
There are three primary ways energy likes to hitch a ride:
- Conduction: This is when energy, particularly heat, moves through a material. It’s like a chain reaction where vibrating particles pass on their extra energy to neighboring particles. It’s how the handle of a metal spoon gets hot when you leave it in a hot soup.
- Convection: This method involves fluid (liquids or gases). Warmer, less dense areas of fluid will rise, and cooler, denser areas will sink. It’s like the circulating currents you see in a boiling pot of water.
- Radiation: Here, energy travels as a wave and doesn’t need a medium (like air or metal) to move through. Sunlight is a prime example – the light and heat from the Sun reach us through the vacuum of space by radiation.
Energy Transfer in Action
Let’s take a game of pool as an example. When you strike a ball with the cue, the kinetic energy (energy of movement) from the cue is transferred to the ball, which then moves across the table. If it hits another ball, some of that energy is transferred again, causing the second ball to move.
Efficiency of Energy Transfer
Not all the energy that starts the journey finishes it. In most real-life situations, some energy is “lost” along the way, often as heat due to friction. However, this isn’t really “lost” – it just ends up somewhere or in a form that we didn’t intend, thanks to the law of conservation of energy, which says energy cannot be created or destroyed, only transferred or transformed.
Types of Energy
Energy comes in many different forms. Here are some of the main types:
Kinetic Energy
Remember when you last kicked a football? That moving football had kinetic energy. It’s the energy of motion – anything that’s moving has kinetic energy. The faster it moves, or the more it weighs, the more kinetic energy it has.
Potential Energy
This is energy that’s stored up, ready to go. It’s the energy an object has because of where it is or its condition. A book on a high shelf has gravitational potential energy because it could fall down. A stretched rubber band has elastic potential energy because it can snap back.
Chemical Energy
This is the energy stored in things like food, fuel, or batteries. It’s released in a chemical reaction. When you eat a sandwich, your body digests it and uses the chemical energy to keep you going.
Thermal (Heat) Energy
This is the energy an object has because of how hot it is. The hotter it is, the more thermal energy it has. When you warm your hands on a mug of hot cocoa, you’re feeling the transfer of thermal energy.
Electrical Energy
This is the energy that electric charges have because of their position or movement. When you plug in your phone to charge, it’s electrical energy that flows from the outlet to your phone battery.
Light Energy
Also known as radiant energy, this is the energy that travels in waves and can move through empty space. It’s the energy we receive from the Sun, and it’s what plants use to grow.
Nuclear Energy
This is the energy stored in the nucleus of an atom. It can be released in nuclear reactions. Nuclear power plants use nuclear energy to produce electricity.
Sound Energy
This is the energy that sound waves carry. When you listen to music, it’s sound energy that travels from the speakers to your ears.
Energy Sources
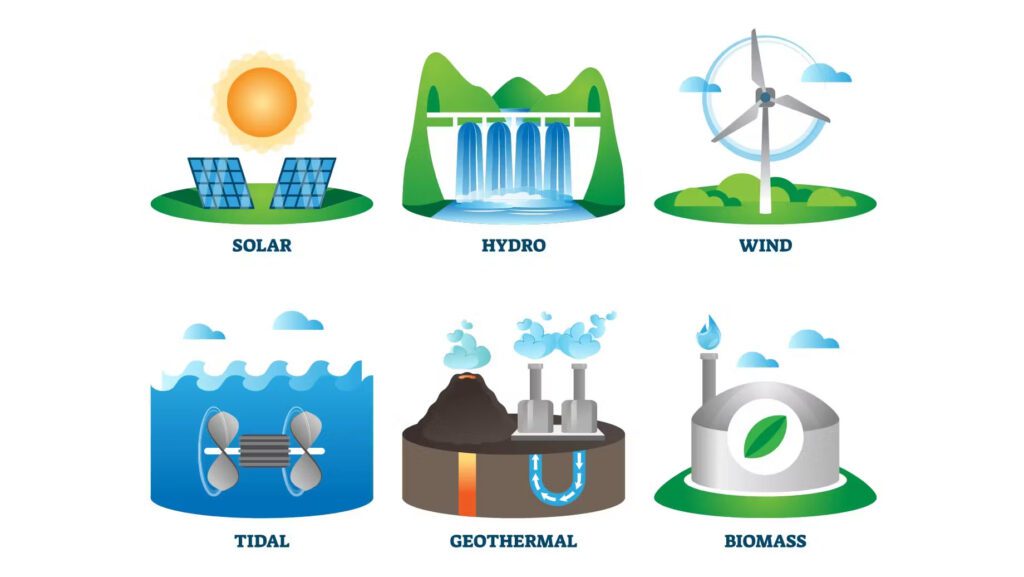
Energy sources can be grouped into two main categories: renewable and non-renewable.
Non-Renewable Energy Sources
These are sources of energy that have a finite supply. They took millions of years to form and cannot be replenished within a human timescale.
1. Fossil Fuels: This group includes coal, oil, and natural gas. They were formed over millions of years from the remains of dead plants and animals, hence the name ‘fossil.’ They’re currently the most widely used energy sources, but burning them releases carbon dioxide, a greenhouse gas that contributes to climate change.
2. Nuclear Energy: This comes from splitting the nuclei of atoms, usually uranium or plutonium, in a process known as nuclear fission. The energy released can be enormous – that’s why it’s used in nuclear power plants. However, it produces radioactive waste, which needs to be stored safely for thousands of years.
Renewable Energy Sources
These are energy sources that can be replenished naturally in a relatively short amount of time.
1. Solar Energy: The Sun is our most abundant energy source. We capture its energy using solar panels, which convert sunlight directly into electricity. This process is silent, pollution-free, and, best of all, the sunlight is free!
2. Wind Energy: Wind turbines convert the kinetic energy of the wind into mechanical energy, which is then transformed into electricity. The wind is an infinite resource, but wind turbines can be noisy and are sometimes seen as visual pollution.
3. Hydroelectric Energy: This is generated by the flow of water. Dams are built to capture the potential energy of water stored at height. When the water is released, it flows down, turning turbines that generate electricity.
4. Geothermal Energy: The Earth’s core is incredibly hot, and we can use this heat as a source of energy. Geothermal power plants use the steam from heated groundwater to turn turbines and generate electricity.
5. Biomass Energy: Biomass is organic material from plants or animals. When burned, the chemical energy in biomass is released as heat. If you’ve ever sat by a wood fire, you’ve experienced biomass energy.
6. Tidal and Wave Energy: These are forms of hydropower that convert the energy obtained from tides and ocean waves into electricity.
Conservation of Energy
What is the Conservation of Energy?
Imagine you’re a magician who can change rabbits into hats and hats back into rabbits. The total number of items you have – rabbits and hats combined – never changes, no matter how much you transform them back and forth. This is the essence of the Conservation of Energy principle.
The principle of Conservation of Energy states that energy cannot be created or destroyed, only transformed from one form into another, or transferred from one object to another. The total amount of energy in a closed system remains constant.
Conservation of Energy in Action
Let’s take the example of a swinging pendulum. When the pendulum is at the highest point of its swing, it’s momentarily still, so it has no kinetic energy (energy of motion). But it has lots of gravitational potential energy (because it’s high up). As it starts to swing down, it loses height, so its gravitational potential energy decreases, but it gets faster, so its kinetic energy increases. When it’s at the bottom of the swing, it’s moving at its fastest, so it has maximum kinetic energy, but it’s at its lowest point, so it has no gravitational potential energy. Then it starts to slow down and rise again, transforming kinetic energy back into potential energy.
So, the pendulum’s energy keeps changing between potential and kinetic, but if you add up the kinetic and potential energy at any point, it always gives the same total. This is Conservation of Energy in action!
Importance of Conservation of Energy
This principle is fundamental in physics. It helps us make predictions and solve problems. It tells us, for example, that if we know how much fuel (chemical energy) a car has, we can calculate how far it can travel (kinetic and potential energy of the moving car) – at least in theory!
However, in real life, no system is perfectly efficient – some energy is always ‘wasted’ as thermal energy (like the heat from your car engine), but it’s not really ‘lost’. It just changes into a form we didn’t want or can’t use. So, the principle of Conservation of Energy still holds!
Sankey Diagrams
What is a Sankey Diagram?
Imagine a city map, where the width of the roads represents the amount of traffic they get. The wider the road, the more cars on it. A Sankey diagram works in a similar way but with energy instead of cars.
A Sankey diagram is a visual representation of energy flows, where the width of the arrows is proportional to the amount of energy. It helps us see how much energy is usefully employed and how much is ‘wasted’, usually as heat.
Sankey Diagrams in Action
Let’s take a lightbulb as an example. We’ll start with 100 units of energy. We draw a wide arrow representing these 100 units going into the lightbulb. However, not all of these 100 units are converted into light. A traditional lightbulb is pretty inefficient, turning only about 5% of its energy into light.
So, in our Sankey diagram, we draw a narrow arrow branching off, representing 5 units going to ‘Light’ (the useful output). The rest of the energy – a whopping 95 units – is ‘wasted’ as heat, which we represent with a much wider arrow labeled ‘Heat’.
By looking at the Sankey diagram, you can instantly see that the lightbulb is inefficient because the arrow for ‘Heat’ is much wider than the arrow for ‘Light’.
Importance of Sankey Diagrams
Sankey diagrams are a powerful tool because they provide a lot of information in a simple and visual way. They give a quick overview of a system’s efficiency by showing where the energy comes from, where it goes, and how much is ‘wasted’.
Changes in energy stores
What Does “Changes in Energy Stores” Mean?
Life is all about changes, and so is energy! Energy can be stored in different ways, and it can move or ‘change’ between these stores.
Think of each type of energy as a different bank account you have. You can move money (energy) between them, but the total amount of money (energy) you have doesn’t change. This is in line with the conservation of energy principle.
Examples of Changes in Energy Stores
Here are a few examples of how energy can change stores:
1. A Skier Going Downhill: When a skier is at the top of a slope, they have lots of gravitational potential energy because they’re high up. As they ski down the hill, that gravitational potential energy decreases, but their kinetic energy (energy of motion) increases because they’re getting faster. So, energy is transferred from the gravitational potential energy store to the kinetic energy store.
2. Eating a Snack: When you eat an apple, your body gets chemical energy from the food. As you digest it, this chemical energy is transferred to different energy stores. Some of it goes into kinetic energy (to move your muscles), some into thermal energy (to keep your body warm), and some into other chemical energy stores (to build and repair cells).
3. Charging a Phone: When you plug in your phone to charge, electrical energy from the outlet is transferred to the chemical energy store in your phone’s battery.
How to Calculate Changes in Energy Stores
Sometimes, you might want to calculate how much energy is transferred between stores. You can use different formulas depending on the types of energy involved. For instance, to calculate the kinetic energy of an object, you can use the formula:
Kinetic Energy = 0.5 * mass * speed^2
Energy and heating
What is the Relationship Between Energy and Heating?
Have you ever rubbed your hands together quickly? You would have noticed that they start to get warm. This is because the mechanical energy of your moving hands is transferred into thermal energy, or heat.
Heating involves transfer of thermal energy from a hotter object to a cooler one. This happens because of a fundamental rule of the universe: energy naturally spreads out if it’s not stopped from doing so.
Examples of Energy and Heating
Here are some daily examples showing the relationship between energy and heating:
1. Boiling Water: When you heat water on a stove, the stove transfers thermal energy to the water molecules, making them move faster and faster until they start to vaporize, turning the water into steam.
2. Running a Laptop: When you use your laptop for a while, you may notice it gets warm, or even hot. This is because electrical energy is not 100% efficiently converted into the light, sound or kinetic energy that you want, and some is ‘wasted’ as thermal energy, which heats up the laptop.
3. Sunlight: The Sun’s light and heat is the result of nuclear reactions taking place in its core. The light energy travels to Earth, and when it hits an object, it’s transformed into thermal energy, heating up the object.
Heat Transfer Methods
Heat can be transferred via three main methods:
1. Conduction: This happens when two objects at different temperatures are in contact with each other; energy is transferred from the hotter object to the cooler one. For example, when you touch a hot mug, the heat is conducted from the mug to your hand.
2. Convection: This is the transfer of heat in fluids (like liquids and gases) where the warmer fluid rises, and the cooler fluid sinks. It’s why we get sea breezes at the beach and it’s how a hot air balloon works.
3. Radiation: This is the transfer of heat through empty space. It doesn’t require a medium like conduction or convection. This is how the Sun’s heat reaches us.
Energy demands
What are Energy Demands?
We live in a world powered by energy. Every device we use, every vehicle we ride, even our own bodies, all need energy to function. This requirement for energy is what we call ‘Energy Demand.’
Factors that Affect Energy Demands
Several factors influence the energy demand of a country or region:
1. Population: More people means more energy is needed for homes, transportation, and services.
2. Economic Activity: Industrial processes, agriculture, and commercial sectors require large amounts of energy. Higher economic activity usually means higher energy demand.
3. Lifestyle: How we live also impacts energy demand. For example, demand for electricity is higher in regions where air conditioning is widely used.
4. Technology: The types of technology we use can increase or decrease energy demand. Energy-efficient appliances reduce the energy needed to perform a task, thereby reducing energy demand.
5. Climate: Regions with extreme temperatures often have high energy demands due to heating or cooling needs.
Why is Understanding Energy Demands Important?
Understanding energy demands is crucial for planning and policy-making. Governments and energy providers need to ensure they can meet the demand at all times. They also need to plan for future energy needs, considering factors like population growth, technological advancements, and climate change.
Work, power and efficiency
What are Work and Power?
Work is a concept in physics that involves applying a force to move an object a certain distance. Imagine pushing a heavy box across the room. The work you’ve done is the force you applied to the box times the distance the box moved. In physics, work is measured in joules (J).
Power is the rate at which work is done or energy is transferred. If you and your friend both push identical boxes across the room, but you do it twice as fast, you’ve exerted more power, even though you both did the same amount of work. Power is measured in watts (W), which is joules per second.
How to Calculate Work and Power
The formula for work is:
Work = Force x Distance
And the formula for power is:
Power = Work / Time
So if you know how much force you applied (in newtons), and how far the object moved (in meters), you can calculate how much work you did (in joules). If you also know how long it took (in seconds), you can calculate your power (in watts).
What is Efficiency?
Efficiency is a measure of how well a system or device converts input energy into useful output. No system is perfectly efficient; some energy is always ‘wasted’ as heat or sound. For example, an incandescent light bulb is only about 5% efficient, because most of the electrical energy it consumes is turned into heat, not light.
Efficiency is usually expressed as a percentage, calculated by:
Efficiency (%) = (Useful Energy Output / Total Energy Input) x 100
So there you have it, a crash course on work, power and efficiency! These concepts help us understand how energy is used and transferred, and inspire us to develop technologies that do this more effectively. So whether you’re sprinting up the stairs, cooking up a storm in the kitchen, or designing the next-generation electric car, remember – it’s all about the work, power and efficiency!
Dissipated Energy
What is Dissipated Energy?
“Dissipated Energy” sounds like your energy after a long day of studying, right? In physics, however, it refers to energy that’s transformed from one form into a form that’s not useful or can’t be easily used.
When energy is transformed, some of it invariably gets converted into forms like heat or sound, that might not be what you wanted. This “wasted” or “unwanted” energy is what we call ‘dissipated energy.’
Examples of Energy Dissipation
Here are some everyday examples of energy dissipation:
1. Toasting Bread: When you toast bread, electrical energy is converted into thermal energy to heat your bread. But you’ll notice the toaster itself also gets hot and the room might even smell like toast. That’s dissipated energy – it doesn’t help toast your bread.
2. Driving a Car: Cars convert chemical energy from fuel into kinetic energy (movement). But a lot of energy is also dissipated as heat from the engine and exhaust, vibrations, and sound (ever wonder why cars get so hot and noisy?).
3. Using a Smartphone: Your phone converts electrical energy from its battery into light, sound, and other forms of energy so you can use apps, take photos, and so on. But some energy is also dissipated as heat, which is why your phone can get warm when you use it a lot.
Importance of Understanding Dissipated Energy
Understanding dissipated energy is crucial for improving the efficiency of our devices and systems. If we know where and how energy is being wasted, we can redesign things to minimize this loss, saving energy and reducing environmental impact.
Energy and Forces
What is the Connection Between Energy and Forces?
In physics, a force is a push or pull that can cause an object to move, stop, or change direction. Energy, on the other hand, is the ability to do work, which includes moving objects — and that’s where forces come in.
A force can change an object’s energy. For example, when you kick a football, you apply a force that gives the ball kinetic energy, sending it flying. Similarly, the gravitational force Earth exerts on the ball can change its kinetic energy by slowing it down (when it’s going upwards) or speeding it up (when it’s coming back down).
Examples of Energy and Forces Interacting
Here are some common examples of how energy and forces interact:
1. Bungee Jumping: When you bungee jump, gravitational force pulls you down, increasing your kinetic energy. As the bungee cord stretches and pulls you back up, the elastic potential energy in the cord increases while your kinetic energy decreases.
2. Compressing a Spring: When you compress a spring, you’re applying a force to it. This gives the spring potential energy, which it can release as kinetic energy when it springs back to its original shape.
3. Friction: When you slide a book across a table, the frictional force between the book and the table opposes the book’s motion, reducing its kinetic energy. This energy doesn’t disappear, though – it’s dissipated as heat.
The Work-Energy Principle
This is a fundamental concept in physics that ties together forces and energy. It states that the work done by all the forces acting on an object equals the change in the object’s kinetic energy.
The formula for this is:
Work Done = Change in Kinetic Energy
This principle helps us understand how forces can change an object’s energy and motion.
Renewable vs Non-renewable Energy Resources
What are Renewable and Non-renewable Energy Resources?
Renewable energy resources are those that can be used over and over again without running out, or they replenish themselves naturally over a short period of time.
Non-renewable energy resources, on the other hand, exist in finite amounts. Once we’ve used them, they’re gone for good, or they take millions of years to replenish.
Examples of Renewable Energy Resources
1. Solar Energy: The sun showers the Earth with more energy in one hour than the entire world uses in a year! Solar panels capture this energy and convert it into electricity.
2. Wind Energy: Wind turbines transform the kinetic energy of the wind into mechanical energy which is then converted into electrical energy.
3. Hydropower: This is energy derived from the movement of water. Dams store water at a height, and when it’s released, it flows past turbines, generating electricity.
4. Biomass Energy: Biomass energy comes from organic materials such as plants and animal waste. This can be burned directly for heat, or converted to biofuels like ethanol and biodiesel.
Examples of Non-renewable Energy Resources
1. Coal: Coal is a black or brownish rock that we burn for energy. It’s been a major energy source for humans for centuries.
2. Oil: This is a liquid fossil fuel that we extract from the ground. We use it to produce gasoline, diesel fuel, jet fuel, and heating oil.
3. Natural Gas: This is a fossil fuel that’s used for heating, cooking, and electricity generation. It’s often found alongside coal and oil.
4. Nuclear Energy: While not a fossil fuel, nuclear energy comes from uranium, a non-renewable resource. Nuclear power plants split uranium atoms in a process called fission to produce energy.
Why Does the Difference Matter?
The use of non-renewable resources has environmental impacts, such as air pollution and climate change. Also, these resources will eventually run out. Renewable resources, on the other hand, are generally much cleaner and will not run out. However, they can be more dependent on conditions like weather and location, and may require large areas of land.
National Grid
What is the National Grid?
The term “National Grid” might sound like a large, steel cage match. But it’s actually more exciting than that (if you’re a physics fan). The National Grid is the network of power stations, substations, and power lines that generate electricity and deliver it to homes, businesses, and other places where it’s needed.
The grid ensures that electricity is transmitted from where it’s produced to where it’s consumed, balancing supply and demand in real time.
How Does the National Grid Work?
1. Generation: Electricity is generated in power stations, using various energy sources such as coal, natural gas, nuclear energy, or renewables like wind and solar.
2. Transmission: The electricity is stepped up to a very high voltage (around 400,000 volts) using a transformer. High voltage is used to transmit electricity over long distances with minimal energy loss.
3. Distribution: When the electricity reaches its destination, it’s stepped down to a lower voltage using another transformer so it can be safely used in homes and businesses.
Why is the National Grid Important?
The National Grid plays a vital role in our daily lives. It ensures that we have a reliable supply of electricity, which powers everything from our fridges and TVs to hospitals and factories. The grid also allows us to use renewable energy from diverse geographical locations, helping us transition to a more sustainable energy future.
Calculating Efficiency
What is Efficiency?
In the world of physics, efficiency isn’t about how quickly you can finish your homework. It’s about how well a system or device converts input energy into useful output.
If you think of a machine as a team, then the input energy is like all the team members, and the output energy is the part of the team that actually gets the job done. The efficiency is the percentage of the team that’s really working!
How to Calculate Efficiency?
We calculate efficiency using a simple formula:
Efficiency (%) = (Useful Energy Output / Total Energy Input) x 100
This formula gives us a percentage that tells us how much of the input energy is used for the intended purpose.
Example of Calculating Efficiency
Let’s say you have a light bulb that uses 100 joules of energy, but only 5 joules is used to produce light. The rest becomes heat, which isn’t what you wanted from your light bulb!
We can calculate the efficiency like this:
Efficiency (%) = (5J / 100J) x 100 = 5%
That means the light bulb is 5% efficient – only 5% of the energy is used to make light, while the rest is ‘wasted’ as heat.
Test Yourself
What does a high specific heat capacity tell us about a substance?
A high specific heat capacity indicates that a substance requires more energy to change its temperature. It can absorb and store a larger amount of heat before it starts to get hot.
If a machine is more efficient, what does that mean about the amount of energy it wastes?
If a machine is more efficient, it means it wastes less energy. More of the energy input is transformed into useful output.
Describe what happens to the kinetic energy of a car when it speeds up.
When a car speeds up, its kinetic energy increases. This is because kinetic energy depends on the speed of the object, with faster objects having more kinetic energy.
Why is geothermal energy considered a renewable energy source?
Geothermal energy is considered a renewable source because it’s powered by the natural heat of the Earth, which is continuously replenished.
How is energy transferred when you stretch a spring?
When you stretch a spring, energy is transferred to the spring’s elastic potential energy store.
How does the National Grid contribute to meeting energy demands?
The National Grid contributes to meeting energy demands by distributing electricity from power stations (where it is produced) to homes and businesses (where it is used).
How does heating a home in the winter relate to energy transfer?
Heating a home in the winter involves energy transfer from a heater (or radiator, or furnace) to the air in the home, increasing the air’s thermal energy.
If an object is lifted higher, what happens to its gravitational potential energy?
If an object is lifted higher, its gravitational potential energy increases. This is because gravitational potential energy depends on height; the higher the object, the more potential energy it has.
What is the advantage of using a renewable energy source like wind power over a non-renewable source like coal?
A renewable energy source like wind power doesn’t run out and produces fewer greenhouse gases, making it more sustainable and less harmful to the environment compared to a non-renewable source like coal.
What kind of energy transformation occurs in a solar panel?
In a solar panel, sunlight (solar energy) is transformed into electrical energy.
What is the unit of energy in the International System of Units?
The unit of energy in the International System of Units is the Joule (J).
Can you define "kinetic energy" and give an example?
Kinetic energy is the energy an object possesses due to its motion. An example could be a moving soccer ball.
What is the difference between a renewable and a non-renewable energy source? Give an example of each.
A renewable energy source can replenish itself naturally in a relatively short time (like solar or wind energy), while a non-renewable energy source cannot and will eventually run out (like coal or natural gas).
What does the Law of Conservation of Energy state?
The Law of Conservation of Energy states that energy cannot be created or destroyed, only transferred or transformed.
Can you explain what a Sankey Diagram is and what it represents?
A Sankey Diagram is a visual representation that shows energy input, useful energy output, and energy that is ‘wasted’ or transformed into less useful forms.
In terms of physics, what does "work done" mean?
“Work done” in physics refers to the process of energy transfer where force causes a displacement. It’s calculated as the product of the force and the distance over which it acts.
How would you define "specific heat capacity", and why is it important?
Specific heat capacity is the amount of heat needed to raise the temperature of 1 kg of a substance by 1 degree Celsius. It’s important because it tells us how different materials will react to heat.
What is "power" in the context of physics and how is it different from "energy"?
Power is the rate at which work is done or energy is transferred, whereas energy is the capacity to do work.
What does "efficiency" mean when discussing energy and how is it calculated?
Efficiency, when discussing energy, refers to how well energy is converted from one form to another. It’s calculated as the ratio of useful energy output to total energy input.
What is "wasted energy" and why does it occur in energy transformations?
“Wasted energy” refers to energy that has been transformed into a form that isn’t useful for the specific process or system. This happens in energy transformations due to unavoidable losses, mainly to the environment in the form of thermal energy (heat).
Additional Knowledge – GCSE Physics – Energy
What is the difference between kinetic energy and potential energy?
The main difference is that kinetic energy is the energy of motion, while potential energy is the stored energy of an object at rest due to its position, shape, or state.
Imagine a roller coaster: at the top of the track, the coaster has lots of potential energy because of its height. As it starts to descend, this potential energy transforms into kinetic energy – the energy of movement. The higher the potential energy (the higher the ride), the greater the kinetic energy (the faster the ride) will be!
Why can’t energy be created or destroyed?
This fundamental principle of physics is known as the Law of Conservation of Energy. The law states that energy cannot be created or destroyed, but only changed from one form into another or transferred from one object to another.
But why is that the case? Let’s break it down.
Understanding the Principle
This principle is tied to the very core of how our universe works. The amount of energy in a closed system (like the universe itself) remains constant over time.
Imagine you’re playing with a set of building blocks. You can rearrange these blocks in all sorts of ways to build castles, cars, or even giant dinosaurs. But no matter what you build, the total number of blocks doesn’t change. You’re not creating or destroying blocks; you’re simply rearranging them.
The same concept applies to energy. The total amount of energy in the universe is like the set of blocks. Energy can be transferred (like passing a block from one hand to the other) or transformed (like rearranging blocks to make a different shape), but the total amount of energy (the total number of blocks) stays the same.
Examples of Energy Transformation
Let’s look at a couple of examples to make this clearer:
1. A falling apple: When an apple falls from a tree, the potential energy it had when it was hanging up high is transformed into kinetic energy as it speeds up towards the ground.
2. A light bulb: When you switch on a light bulb, the electrical energy from the power grid is transformed into light and heat energy in the bulb.
In both examples, the total amount of energy remains constant; it just changes form.
What is ‘work done’ in terms of physics?
What is ‘Work Done’ in Physics?
In everyday life, work might mean your homework, your chores, or your job. But in physics, “work” has a very specific meaning. It refers to the process of energy transfer caused by a force.
In simple terms, when you apply a force to move an object a certain distance, you’re doing work on the object. So, you’re doing “work” in the physics sense when you’re lifting weights, pushing a shopping cart, or even just picking up a book!
How is Work Done Calculated?
The amount of work done (W) is calculated by multiplying the force applied (F) by the distance over which it’s applied (d), but only the force in the direction of the movement counts. The formula is:
W = Fd
and it’s measured in Joules (J).
Example of Work Done
Suppose you’re dragging a suitcase that weighs 15 kg a distance of 10 meters. You’re pulling the suitcase with a force of 20 Newtons. The work done would be:
W = Fd = 20N * 10m = 200 Joules
That means you’ve transferred 200 Joules of energy to move the suitcase.
Remember, in physics, no work is done if there’s no movement. So, if you’re pushing against a wall and it doesn’t move, you’re not doing any “work” in the physics sense. You might be sweating, but that’s a whole other kind of work!
So, the next time someone asks if you’ve done your work, you can tell them about that heavy door you had to push open – and how much ‘work’ you did in the process! Physics work, of course!
What is power and how is it different from energy?
What is Power?
In physics, Power is not about who’s in charge. It’s about how quickly work is done or energy is transferred. If Energy is the total amount of work done, then Power is the speed at which that work is done.
It’s like the difference between a sprinter and a marathon runner. The sprinter (high power) can cover a short distance very quickly, while the marathon runner (lower power) covers a much longer distance but at a slower pace. Both are ‘doing work’, but at different rates.
The formula to calculate power (P) is:
P = Work done (W) / Time taken (t)
It’s measured in Watts (W), named after the Scottish engineer James Watt.
How is Power Different from Energy?
While energy refers to the capacity to do work, power refers to the rate at which that work is done.
Think of it like this:
Energy is like the amount of fuel in your car’s tank. It represents how far your car could potentially go.
Power, on the other hand, is like the car’s horsepower. It represents how quickly your car can use that fuel to go a certain distance.
Example of Power
Let’s say you and your friend are having a competition to see who can carry a 10 kg box up a flight of stairs the fastest. You both do the same amount of work (you’re moving the same box up the same stairs), but if you make it to the top first, you’ve demonstrated greater power. You’ve done the same amount of work in less time.
Why is some energy ‘wasted’ in energy transformations?
What is Wasted Energy?
In the world of physics, when we say energy is “wasted”, it doesn’t mean it disappears. Remember, energy can’t be created or destroyed (thanks, Law of Conservation of Energy!). Instead, “wasted energy” is energy that has been transformed into a form that’s not useful for the purpose at hand.
It’s like going to a concert, but instead of listening to the band, you spend the whole time stuck in line for the bathroom. The concert (energy) is still happening, but it’s not being used for the purpose you wanted (listening to the band) – that’s the energy being “wasted”.
Why is Energy ‘Wasted’ in Energy Transformations?
During any energy transformation, some energy is always “wasted” because it’s transformed into forms like sound or heat, which may not be the desired forms for that particular process. This is mainly due to the forces of friction and air resistance.
For example, in a car engine, the burning fuel (chemical energy) is meant to be converted into kinetic energy to move the car. But some of this energy also transforms into heat and sound energy (ever noticed how an engine gets hot or makes noise?). These forms of energy don’t help the car move, so they’re considered ‘wasted’ in this context.
The Impact of Wasted Energy
The more energy a machine wastes, the less efficient it is. That’s why engineers are always trying to design machines and systems to minimize energy wastage and improve efficiency.
What is specific heat capacity and how is it used?
What is Specific Heat Capacity?
In the world of physics, “Specific Heat Capacity” (often just called specific heat) is a bit like a material’s thermal personality. It tells us how a certain substance likes to heat up or cool down.
More technically, it’s the amount of heat energy needed to raise the temperature of 1 kilogram of a substance by 1 degree Celsius (or Kelvin). It’s a measure of how much heat energy a substance can hold before its temperature changes.
How is Specific Heat Capacity Used?
Specific heat is a crucial concept in understanding how different substances behave under heat. It’s used in various areas from cooking to climate science!
Imagine you’re cooking pasta. You put the water (high specific heat) on the stove, and it takes some time to heat up. But the metal pot (low specific heat) heats up really quickly! This difference is all down to their specific heat capacities.
Or think about our planet. The oceans (again, high specific heat) absorb lots of heat without their temperature going up much, moderating our climate. But the land (lower specific heat) heats up and cools down much more quickly, creating the variety of climates we see on Earth.
The specific heat capacity is also used in calculations involving heat transfer and in designing heating and cooling systems.
Formula and Units
The formula for calculating heat energy (Q) is:
Q = mcΔT
where:
- m is the mass of the substance,
- c is the specific heat capacity, and
- ΔT is the change in temperature.
The unit of specific heat in the International System of Units (SI) is joule per kilogram per kelvin (J/kg·K) or joule per kilogram per degree Celsius (J/kg·°C).
What are Sankey diagrams used for in the study of energy?
Sankey Diagrams are used to visually represent energy transfers and transformations in a system. They help us see the total energy input, the useful energy output, and the ‘wasted’ energy (usually as heat) in one clear picture.
They’re kind of like energy detectives, showing us where energy gets ‘lost’ (or transformed into less useful forms) and where it’s most effectively used.
Examples of Sankey Diagrams Usage
Let’s say we want to represent the energy use in a light bulb. The total electrical energy going into the bulb would be shown by a wide arrow. Then, two arrows would branch out: a narrower one for the useful light energy output, and a wider one for the heat energy (which is usually considered ‘wasted’ in this case).
This diagram helps us see that the bulb transforms most of the electrical energy into heat, not light, making it not very efficient. And that’s why many people now prefer LEDs, which have a much better ‘Sankey score’!
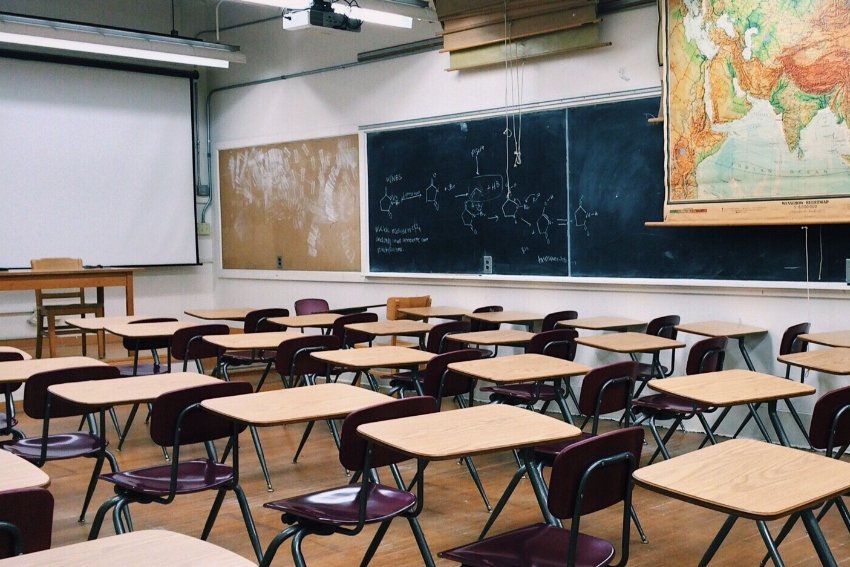