What are Power and Energy?
Energy in physics is the ability to do work or the capacity to cause change. Let’s say you’re a super fit runner who has decided to run up a hill. The energy you use to reach the top of the hill is like the electrical energy a device uses to function. The harder the task, the more energy you need. A light bulb, for instance, requires less energy compared to an electric heater because lighting up a small bulb is easier (requires less work) than heating a room.
Now, imagine you have two runners: Runner A sprints up that hill, while Runner B walks up leisurely. They both do the same amount of work (or use the same amount of energy) to reach the top of the hill, but the time they take is different. Here’s where Power comes in.
Power is the rate of using energy or the amount of work done per unit time. If you think of our runners, they both used the same energy, but Runner A demonstrated more power because they used their energy faster.
In terms of electricity, power (measured in watts) is calculated by multiplying the voltage (volts) by the current (amperes). For example, a laptop charger might have a power rating of 65 watts, which means it uses 65 joules of energy every second when it’s working at maximum capacity.
Energy consumption is then calculated by multiplying power (watts) by time (seconds).
E = P x t/1000
For instance, if you leave a 10-watt LED light bulb on for an hour (3600 seconds), it will consume 10 watts x 3600 seconds = 36,000 joules (or 0.01 kilowatt-hours) of energy.
Remember, devices with higher power ratings (like a gaming console) use more energy in a shorter time, just like a runner sprinting up a hill, while devices with lower power ratings (like an LED bulb) use less energy over the same period, similar to a person walking up the hill. So, it’s more energy-efficient (and cost-effective!) to use lower-power devices whenever possible.
Electrical Circuit
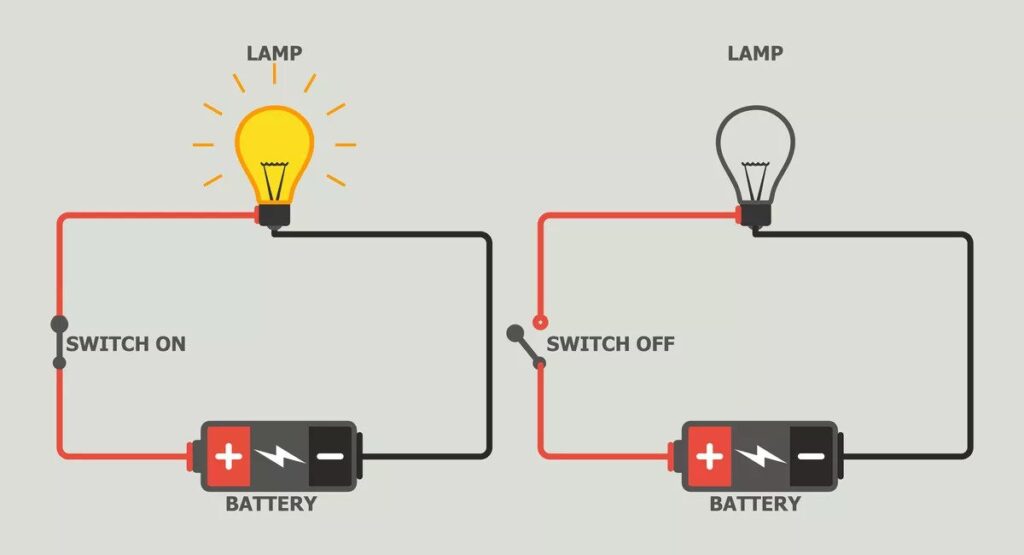
In an Electrical Circuit, energy is transferred from a power source (like a battery) to various components (like a light bulb or a resistor).
Here’s how it works:
Battery/Power Source
This is like your body’s food. Just as food gives your body energy to function, the battery provides electrical energy to the circuit. The battery converts its stored chemical energy into electrical energy.
Wires
Think of these as your body’s blood vessels. Just as blood vessels transport nutrients and energy to different parts of your body, the wires carry electric charge (and therefore energy) around the circuit.
Components (explained soon)
These are like your body’s muscles. When you exercise, your muscles use the energy carried by your blood to do work (like lifting weights or running). Similarly, in a circuit, the electrical components use the electrical energy carried by the wires to do work. For instance, a light bulb converts electrical energy into light and heat.
Closed Circuit
For this whole system to work, it must be a closed loop, meaning there’s a continuous path for the electric charges to flow. This is like your body’s circulatory system – blood must have a continuous path to flow, or else your body can’t function.
Remember, the goal of a circuit is to transfer energy from the source to the components that will convert it into other forms of energy like light, heat, or mechanical energy. When you turn on a light switch, for example, you’re closing an electrical circuit and allowing energy to flow from the power source to the light bulb, illuminating your room.
Components
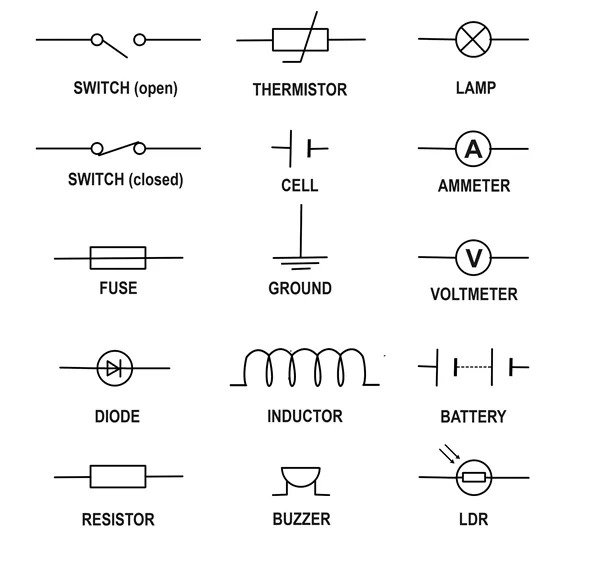
Resistor
Think of a resistor like a school hallway packed with students. If there’s a test coming up and everyone’s trying to get to the library, the crowded hallway is going to slow them down, right? In a similar way, a resistor resists or limits the flow of electric current in a circuit. It’s like the traffic controller of the electrical world!
Fixed Resistor
This is like our school hallway at a fixed width – it can only accommodate a certain number of students (current) at a time. No matter how much more you try to push, it simply can’t handle more than its capacity.
Variable Resistor
Now, imagine if you could magically adjust the width of that hallway. More space – more students can pass through. Less space – fewer students pass. This is exactly what a variable resistor does. It can be adjusted to increase or decrease the resistance, therefore controlling the amount of current that can flow.
Switch
A switch is like a door. When you open the door (switch ON), students can go through the hallway (current flows). When you close the door (switch OFF), no one can pass (current stops).
Lamp (Light Bulb)
Think of a light bulb like the school’s football star – it takes the energy (electricity) and transforms it into something (light). When current passes through the filament of the light bulb, it gets so excited (hot) that it starts to glow, illuminating our surroundings!
Battery
A battery is like the school’s cafeteria. Just as students get their energy from the food at the cafeteria, electrical devices get their energy from the battery. It provides the push (voltage) needed to make the current flow in a circuit.
What Carries the Useful Energy from a Lamp to Its Surroundings?
The useful energy from a lamp is primarily carried to its surroundings in the form of light and heat. When a lamp is switched on, electrical energy is converted into light energy, which illuminates the surrounding area. This light energy enables us to see and perform activities in the illuminated space.
Additionally, a significant portion of the electrical energy is also converted into heat energy. This heat is dispersed into the surroundings, warming the air around the lamp. The combination of light and heat energy radiates outward from the lamp, making it a useful source of both illumination and warmth in various settings.
How do Transformers work?
Picture yourself using a pair of walkie-talkies with your friend. Your friend’s voice is too low, and you can’t hear them properly. What do you do? You turn up the volume, right? That’s basically what a transformer does to electric current – it can turn up (step up) or turn down (step down) the voltage.
A transformer consists of two coils of wire wrapped around an iron core. These two coils are not directly connected; instead, they’re linked magnetically through the core. Here’s how it works:
Input Voltage
The first coil (primary winding) receives the input voltage. When the AC power source is connected to this coil, it produces a changing magnetic field around the iron core.
Magnetic Induction
The second coil (secondary winding) is exposed to this changing magnetic field, and due to electromagnetic induction, a voltage is produced in this coil.
Output Voltage
The voltage that we get from the secondary coil is the output voltage.
Stepping Up or Stepping Down
Whether a transformer is a step-up or step-down transformer depends on the number of turns in the primary and secondary coils.
If the secondary coil has more turns than the primary coil, the output voltage will be higher than the input voltage. This is a Step-Up Transformer and works like increasing the volume on your walkie-talkie. It’s used in power stations to raise the voltage for transmission over long distances.
If the secondary coil has fewer turns than the primary coil, the output voltage will be lower than the input voltage. This is a Step-Down Transformer, and it’s like lowering the volume on your walkie-talkie. These are used in various places like laptop chargers and phone chargers to reduce the voltage to a safe level for use.
So, in a nutshell, transformers are like the volume controls of electricity – they adjust the voltage to the right level for our needs!
Three-pin plugs
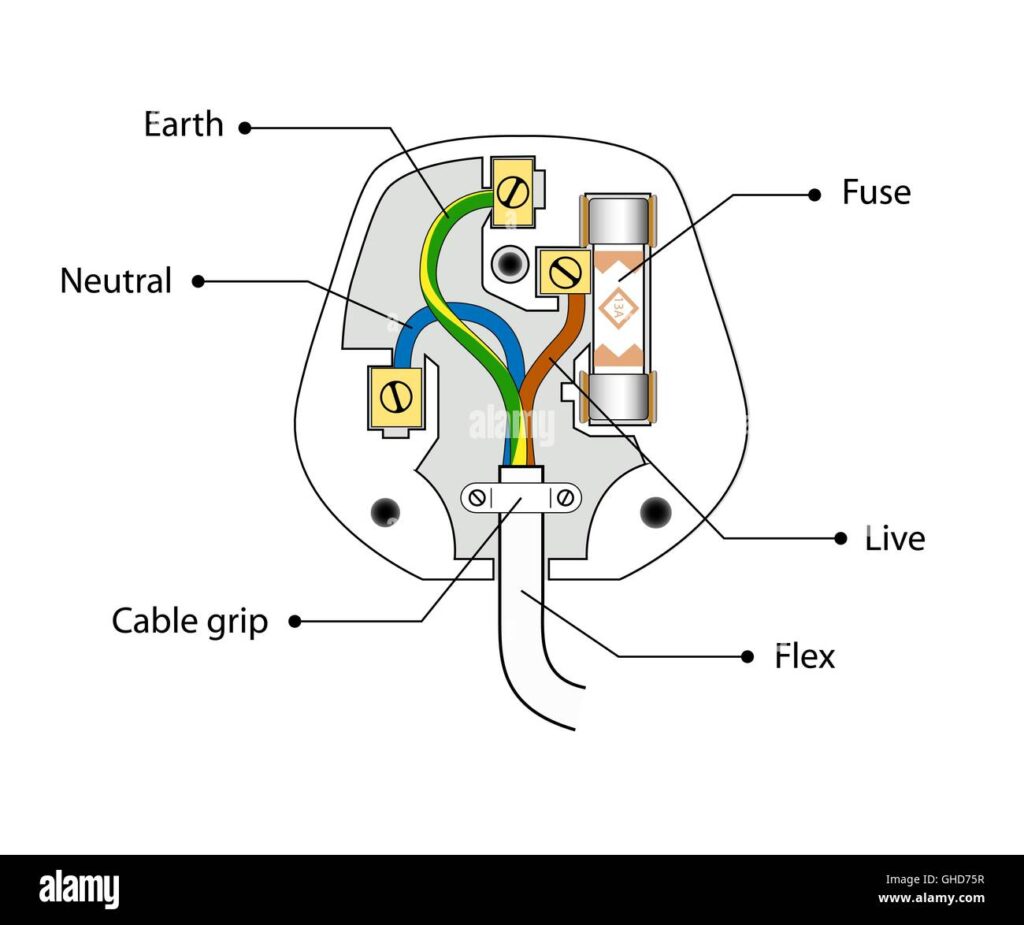
A three-pin plug connects an electrical device to the electricity supply. Each of the three pins has a specific function:
Live Pin
This is the pin that carries electricity into the appliance from the power source. Think of it as the front door of a busy restaurant – it’s where all the customers (electricity) come in. The live pin is usually on the right and is slightly shorter than the earth pin. It’s also fused for safety purposes. The voltage on the live wire is around 230 volts in the UK.
Neutral Pin
This is where electricity leaves the appliance after it has done its job. Back to our restaurant analogy, it’s the back door where customers leave after they’ve had their meal. The neutral pin is typically on the left and is longer than the live pin but shorter than the earth pin.
Earth Pin
This is a safety feature. If something goes wrong with the appliance (like a wire coming loose and touching the metal case), the earth pin provides a path for the electricity to flow safely back to the ground instead of giving you a shock. In our restaurant, the earth pin is like the emergency exit – it’s not used unless something goes wrong. The earth pin is the longest and is at the top when the plug faces upwards.
Inside the plug, there’s also a fuse between the live pin and the live wire. The fuse is another safety device. If too much current flows into the appliance (maybe because of a fault), the fuse ‘blows’, or melts, breaking the circuit and stopping the flow of electricity. This protects the appliance and prevents fires.
What is Ohm’s Law?
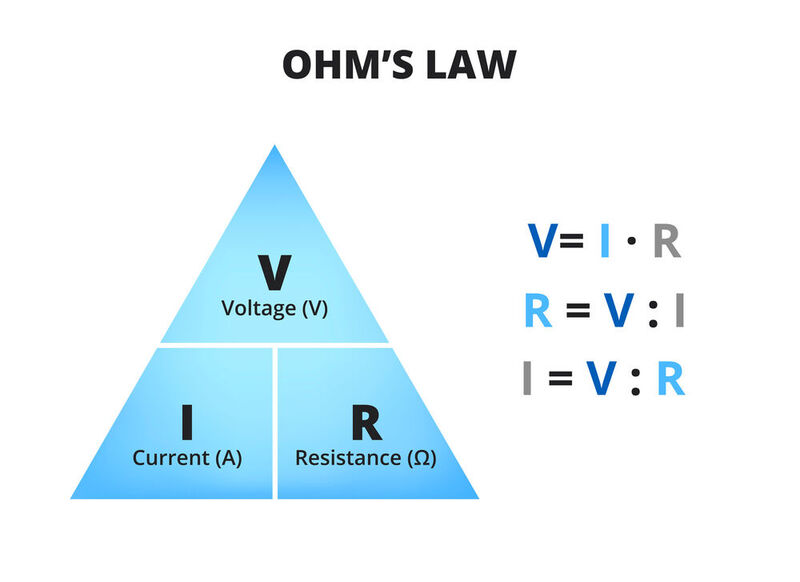
Ohm’s Law is named after the German physicist, Georg Simon Ohm, who first formulated it in 1827. It’s a principle that gives us a relationship between three fundamental electrical quantities: voltage, current, and resistance.
In simple terms, Ohm’s Law states that the current flowing through a conductor between two points is directly proportional to the voltage across the two points, and inversely proportional to the resistance between them.
Sounds a bit complex, right? Let’s break it down with an analogy:
Imagine you’re in a park, and you see a long, wide slide. The height of the slide is like the voltage (V) – it’s the “push” that gets you going. The width of the slide represents the resistance (R). A wide slide lets more people (current, I) slide down at the same time, while a narrow slide lets fewer people slide down. According to Ohm’s Law, if you increase the height of the slide (voltage), more people will be able to slide down (current increases). However, if you make the slide narrower (increase resistance), fewer people will be able to slide down (current decreases).
Mathematically, Ohm’s Law is often written as:
V = I x R
- V is the voltage across the conductor (measured in volts)
- I is the current through the conductor (measured in amperes, or amps)
- R is the resistance of the conductor (measured in ohms, Ω)
So if you know any two of voltage, current, and resistance, you can find the third using Ohm’s Law. This makes it a powerful tool in the world of electronics and electricity!
Just remember, like any law, Ohm’s Law only applies under certain conditions. For example, the material of the conductor needs to maintain a constant temperature for Ohm’s Law to hold true. Real-world materials may not always obey Ohm’s Law perfectly, but it’s still a good starting point for understanding the relationships between voltage, current, and resistance.
Kirchhoff’s Current Law (KCL)
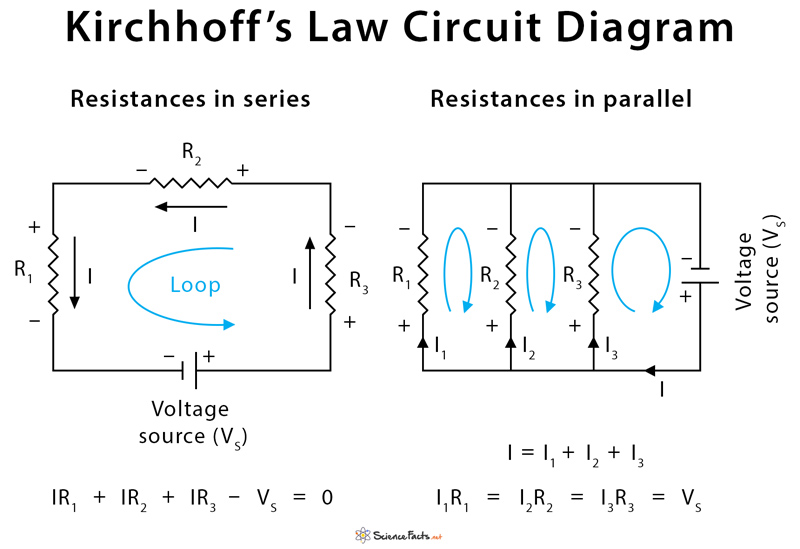
KCL is like the principle of conservation of crowd in a music festival. Imagine several entrance gates (branches) where people (electric current) enter into the festival (node/junction). Now, if nobody is hiding in the toilets or leaving the festival, the total number of people who entered the festival would be the same as the total number of people inside the festival.
So, Kirchhoff’s Current Law says that the total current or charge entering a junction or node in a circuit must be the same as the total current or charge leaving the node.
Kirchhoff’s Voltage Law (KVL)
This rule can be thought of as a fun roller coaster ride. At the start of the ride, the roller coaster car is lifted to a high point (this is like the battery giving energy or voltage to the charges). As the car races around the track (the circuit loop), it loses some of its height (voltage) with each twist and turn (resistor) it encounters. By the time the car returns to the start (completes the circuit loop), it’s used up all the height (voltage) it started with.
So, Kirchhoff’s Voltage Law states that the total voltage supplied in a closed loop of a circuit is always equal to the total voltage drop across the resistances in that loop. In equation form, it’s:
∑V_supply = ∑V_drop
These two laws are incredibly powerful tools for analysing complex circuits. They provide the foundation for techniques like nodal analysis and mesh analysis, which are used by electrical engineers to solve for unknowns in circuits.
AC and DC
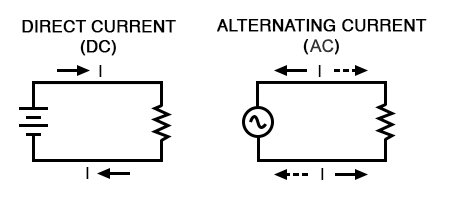
1. Direct Current (DC)
Direct Current is like a one-way road. The electric charge (or “current”) flows in one constant direction. Imagine you’re walking straight along a path without turning back – that’s how DC works!
DC is the type of electricity that comes from sources like batteries. It’s also the type of electricity that most electronic devices need to function. That’s why many devices come with an adapter, which converts the AC from the wall socket into DC.
2. Alternating Current (AC)
Alternating Current, on the other hand, is like a zigzag path. The electric charge doesn’t flow in one constant direction, but instead keeps reversing back and forth. It’s like if you were walking along a path but kept changing your direction every few seconds.
The electricity supplied to homes and businesses from the power grid is AC. One of the reasons AC is used is because it can be easily transformed to high voltages for transmission over long distances, and then stepped down again for safe use in homes and businesses.
A big difference between AC and DC involves voltage. With DC, the voltage is constant, like the speed of a car driving straight down a road. With AC, the voltage is continuously varying, like a car that’s always accelerating and decelerating.
Safety Precautions
Here are some essential safety precautions when working with electrical circuits:
- ❌ Never touch electrical outlets or appliances when your hands are wet. Water is a good conductor of electricity. Touching electrical appliances with wet hands can lead to electrical shocks.
- ❌ Always use insulated tools. When working with electrical circuits, always use tools with insulated handles. This can prevent the current from passing through you if you accidentally touch a live part of the circuit.
- ❌ Unplug before servicing. If you’re working on an appliance or device, always make sure it is unplugged before you start.
- ❌ Beware of damaged cords and plugs. Damaged cords can expose wires, creating a risk of shock, burns, or fire. Replace any damaged cords immediately.
- ❌ Don’t overload outlets. Overloading an outlet can lead to overheating and potentially cause a fire.
- ❌ Always have a qualified professional do major electrical work: Even if you’re a DIY enthusiast, some jobs should be left to the professionals. Electrical work can be dangerous and is often governed by codes and regulations.
- ❌ Use appropriate safety equipment. Safety glasses, gloves, and appropriate clothing should be worn when working with electrical components.
- ❌ Familiarise yourself with the location of the main switch or circuit breaker. Knowing how to turn off the entire electricity supply quickly can be critical in case of an emergency.
- ❌ Understand symbols and labels. Electrical devices and components use symbols and labels to indicate important safety information. Make sure you understand what these mean.
- ❌ Stay away from power lines. Always keep yourself, your equipment, and any materials at least 10 feet away from power lines.
Remember, when it comes to electricity, safety should always be your first priority!
Uses of Static Electricity
Photocopiers and Laser Printers. These machines use static electricity to transfer toner to the paper and then keep it in place before it’s permanently attached.
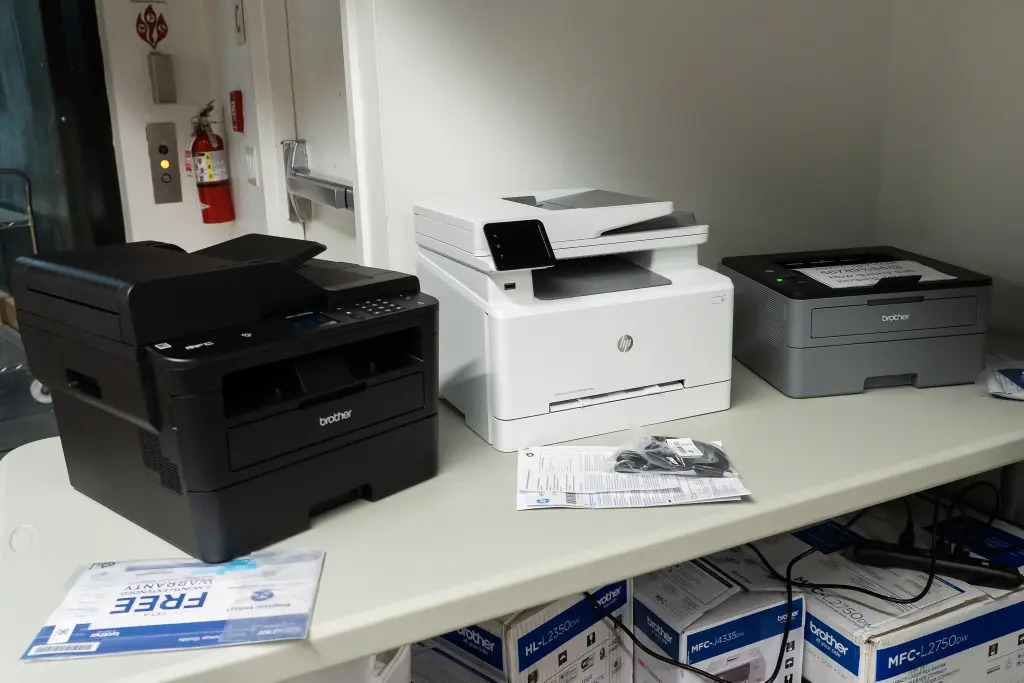
Paint Sprayers. Car manufacturers often use static electricity to help give cars a uniform paint job. The car body is given a positive charge, and the paint is given a negative charge. This ensures that the paint particles are attracted evenly across the car’s body.
Dust Removal. Air purifiers often use static electricity to remove dust particles from the air. The dust particles pick up a charge as they pass through the electric field and are then attracted to plates with an opposite charge.
Insect Control. Bug zappers use static electricity to attract and then kill insects.
Dangers of Static Electricity:
- ⚠️ Fuel Sparks. Static electricity can be a real danger when filling up with gasoline. The spark generated from static electricity can ignite the gasoline vapors, causing a fire or explosion.
- ⚠️ Damage to Electronics. Many electronic components are sensitive to static electricity and can be damaged if not handled properly. That’s why workers wear anti-static wristbands when assembling electronic devices.
- ⚠️ Static Shocks. While these are generally harmless and more surprising than dangerous, they can still be unpleasant.
- ⚠️ Inflammable Environments. In environments where inflammable substances are handled, a static discharge can ignite vapors and cause explosions.
- ⚠️ Lightning Strikes. A lightning strike is a large-scale natural example of static discharge. While the chances of being struck by lightning are quite low, the results can be deadly.
Insulators and Incubators
1. Conductors
Conductors are materials that allow electric charges to move freely. They have a sea of free electrons (negatively charged particles) that can drift, or flow, in the presence of an electric field. This flow of electrons is what we call electric current.
For example, imagine a busy highway with lots of cars (electrons) that can easily move from one place to another. This is what’s happening in conductors.
Common conductors include most metals. Copper is often used in electrical wires because it is a great conductor and is relatively inexpensive. Gold, while more expensive, is used in some electronic applications because it is very resistant to corrosion.
2. Insulators
Insulators, on the other hand, are materials that impede the free flow of electric charges. They have tightly bound electrons that cannot move around very much.
To use the highway analogy again, imagine a road with lots of speed bumps and roadblocks. Cars (electrons) can’t move around as easily. This is what’s happening in insulators.
Common insulators include rubber, glass, plastic, and dry wood. Insulators are used to protect us from the dangerous effects of direct contact with electrical wires and components.
It’s important to note that no material is a perfect conductor or insulator. Even great conductors have some resistance to the flow of electricity, and insulators will conduct electricity if the electric field is strong enough. However, this categorization gives us a useful way to understand how different materials react in the presence of electricity.
3. Semiconductors
There’s a third category worth mentioning: semiconductors. These materials can sometimes act like conductors and sometimes act like insulators. Silicon, for example, is a common semiconductor used in electronics. The conductivity of semiconductors can be controlled with impurities and temperature, which is why they’re so useful in technological devices.
Charging By Friction
“Charging by Friction” is the process where objects are electrically charged by rubbing or contact. When two different materials are rubbed together, electrons may transfer from one material to the other. This can leave one object with a positive charge (having lost some electrons) and the other with a negative charge (having gained those electrons).
Let’s break down how this works:
Friction causes electron transfer. When you rub two objects together, the friction between them can cause electrons to be stripped away from one object and transferred to the other.
Electron affinity determines direction. The direction of electron transfer depends on the nature of the materials involved. Some materials have a higher “affinity” for electrons (they’re more eager to take electrons) than others.
Objects become charged. The object that gains electrons becomes negatively charged, and the object that loses electrons becomes positively charged.
A common example of charging by friction is rubbing a balloon against your hair. If you’ve ever done this, you’ll notice your hair standing on end. This is because your hair loses electrons to the balloon, becoming positively charged, and each individual hair repels the others (because like charges repel). At the same time, the balloon, now negatively charged, can stick to walls or other objects because it’s attracted to their positive or neutral charge.
This principle of charging by friction is also the basis of static electricity. But remember, while these static shocks are generally harmless, in certain situations (like near flammable gases), they can be dangerous as the spark can ignite the gas and cause an explosion. So, it’s always important to understand and respect the power of electricity, even on a small scale.
Coulomb’s Law
In a nutshell, Coulomb’s Law describes the force between two charges. It’s named after French physicist Charles-Augustin de Coulomb, who published it in the 18th century.
Coulomb’s Law states that the force (F) between two electrical charges is directly proportional to the product of their charges (q1 and q2) and inversely proportional to the square of the distance (r) between them. It’s also dependent on a constant (k), which is known as Coulomb’s constant.
In mathematical terms, this law is expressed as:
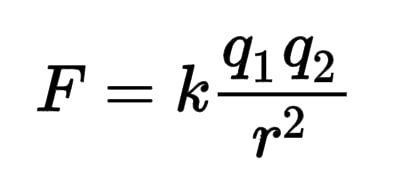
Where:
- F is the magnitude of the force between the charges
- q1 and q2 are the amounts of charge
- r is the distance between the charges
- k is Coulomb’s constant, approximately equal to 8.99 × 10⁹ N.m²/C²
Here’s what all this means in simpler terms:
- If the charges are the same sign (both positive or both negative), the force between them is repulsive – they push each other away.
- If the charges are of different signs (one positive, one negative), the force between them is attractive – they pull towards each other.
- The larger the charges, the stronger the force between them.
- The greater the distance between the charges, the weaker the force.
An easy way to visualise Coulomb’s Law is to think of charges as if they were people on a dance floor. The more “popular” (more charge) a person is, the more people (other charges) want to dance with them (more force). But if two people have a feud (like charges), they’ll want to stay far away from each other (repulsion). Finally, the further apart the people are, the less likely they’ll interact (force decreases with distance).
Coulomb’s Law is a cornerstone of electrostatics and is crucial to many areas of physics and engineering. It enables us to understand how charges interact and how electrical fields are created, leading to the technology we use every day.
Electric Fields
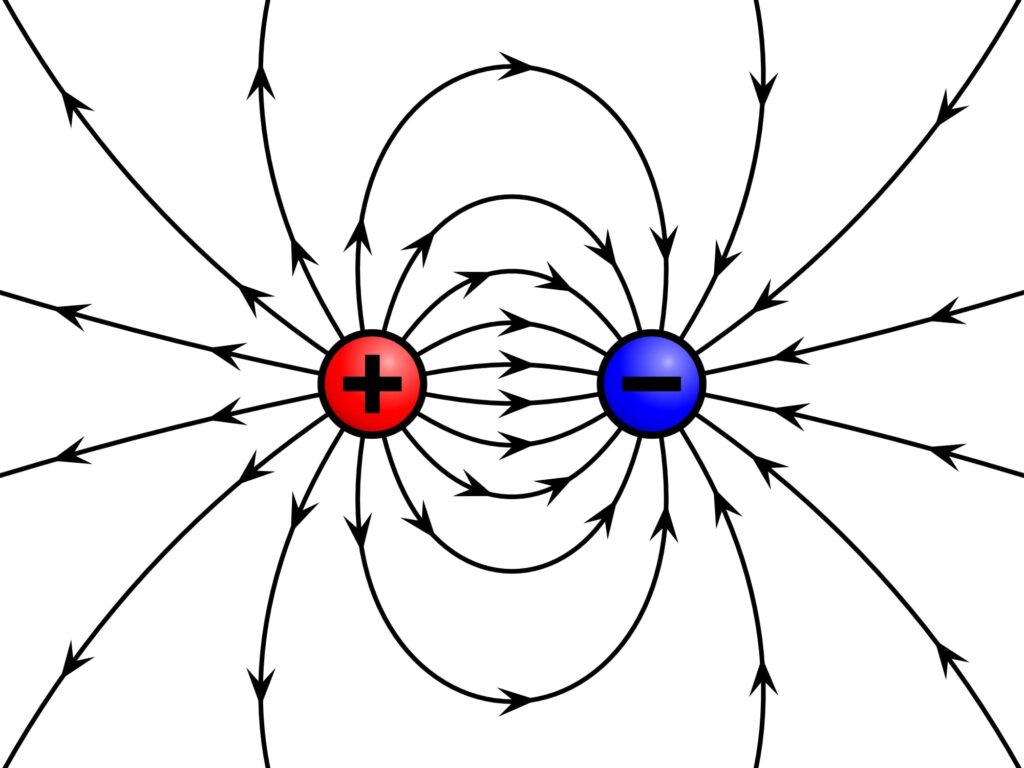
An electric field is an invisible force field that exists around any electrically-charged object. It describes how an electric charge influences the region around it. The direction of the field is taken to be the direction of the force it would exert on a positive test charge.
Here’s how to think about it:
Imagine you’re at a party, and there’s a really popular person in the room – let’s say they’re the electric charge. Now, the way they influence the mood and behaviour of other people in the room, that’s the electric field. People close to them are more affected (stronger field), and people further away are less influenced (weaker field).
Now, onto the more technical aspects:
Field Direction
The electric field direction is defined as the direction that a positive test charge would move if it was dropped in the field. So, the field direction is always away from positive charges and towards negative charges.
Field Strength
This is a measure of the force experienced by a positive test charge in the field. The closer the test charge is to the source charge, the stronger the force, and hence, the stronger the electric field.
Field Lines
Electric field lines are imaginary lines that represent both the direction and the strength of the electric field. The lines point in the direction of the field (the direction a positive test charge would move), and the denser the lines, the stronger the field.
Electric fields are super important in many areas of physics and engineering, especially in the design and functioning of electrical equipment. For instance, the concept of an electric field is central to the operation of capacitors, a key component in many electronic devices.
A Magnetic Field
A magnetic field is a region around a magnetic material or a moving electric charge within which the force of magnetism acts. It’s an invisible field that exerts a force on substances that are susceptible to magnetism and is detected by the force it exerts on moving electric charges.
Magnetic Fields Around Magnets
Every magnet has a magnetic field around it. This is why magnets can push or pull other magnets or magnetic materials (like iron) without touching them – the magnetic field is at work!
You can visualise a magnetic field by placing a magnet underneath a piece of paper and sprinkling iron filings on top. The filings align along the field lines, allowing you to see the field’s shape. It emerges from the magnet’s north pole and enters the south pole, forming a closed loop.
Magnetic Fields Around Electric Currents
When electric charge moves, as it does in a wire with an electric current, it generates a magnetic field around it. The right-hand rule can help you determine the direction of the magnetic field. If you point your right thumb in the direction of conventional current (from positive to negative), your fingers curl in the direction of the magnetic field.
Field Strength
The strength of a magnetic field decreases with distance from the magnet or current that’s causing it. Near a strong magnet or a large current, the magnetic field can be quite powerful, but it quickly weakens as you move away.
Earth’s Magnetic Field
Our Earth itself acts like a huge bar magnet with a magnetic field surrounding it, called the geomagnetic field. This field is why compasses work – the compass needle aligns with the Earth’s magnetic field and points towards the magnetic North and South poles.
Electromagnetism
Magnetic fields are integral to electromagnetism, the branch of physics involving the study of the electromagnetic force, a type of physical interaction that occurs between electrically charged particles.
Understanding magnetic fields is essential for many technologies, including electric motors, generators, MRI machines, and even computer data storage!
What is Electromagnetic Induction?
Electromagnetic induction is the process of inducing (or creating) a voltage (or electromotive force) across a conductor when it is exposed to a varying magnetic field. This process can also induce an electric current if the circuit is closed.
The principle of electromagnetic induction was discovered by Michael Faraday in 1831, and it consists of two primary laws:
Faraday’s Law of Induction
Faraday’s law states that the induced electromotive force in any closed circuit is equal to the negative of the rate of change of the magnetic flux through the circuit. Simply put, the faster the magnetic field changes, the more voltage is induced in the conductor.
Lenz’s Law
Lenz’s law is a way to understand direction of the induced current. It states that an induced electromotive force (emf) always generates a current that creates a magnetic field that opposes the initial changing magnetic field.
Practical Applications of Electromagnetic Induction:
Electric Generators
An electric generator works by rotating a coil within a magnetic field, changing the magnetic field through the coil and inducing an electric current.
Transformers
Transformers use electromagnetic induction to increase or decrease the voltage of alternating current (AC). They consist of two coils, primary and secondary, wound around a magnetic core. When alternating current passes through the primary coil, it creates a changing magnetic field, which then induces a voltage in the secondary coil.
Wireless Charging
Devices like electric toothbrushes or smartphones use electromagnetic induction for wireless charging. The charger has a coil with an alternating current passing through it. This creates a changing magnetic field, which induces a current in the coil of the device, thereby charging its battery.
Induction Stovetops
These stovetops heat pots and pans via electromagnetic induction. An alternating current in the stovetop’s coil generates a changing magnetic field, inducing an electric current directly in the pot (which needs to be made of a ferromagnetic material), which then heats up due to electrical resistance.
So, the concept of electromagnetic induction is fundamental to modern life, from the generation of electricity that powers our homes, to many everyday gadgets and appliances.
Test Yourself
What is the primary difference between AC and DC current?
AC current alternates its direction periodically, while DC current flows in a single direction.
Can you draw a basic circuit diagram including a battery, a resistor, a switch and a bulb?
Answers may vary but should include a power source (battery), load (light bulb), switch, and resistor all connected in a loop with wires.
What is Ohm's Law, and how can you use it to calculate the current flowing through a 10-ohm resistor connected to a 20-volt power supply?
Ohm’s Law states that V = IR. By rearranging it to I = V/R, we can find that the current is 20V/10Ω = 2 Amps.
How does a transformer work, and what are the uses of step-up and step-down transformers?
A transformer works on the principle of electromagnetic induction. A step-up transformer increases the voltage while decreasing the current, and a step-down transformer decreases the voltage while increasing the current. They are used in power transmission and electronic devices respectively.
Why is the live wire dangerous to touch, even when the switch is turned off?
The live wire carries the current from the power source. Even when the switch is off, the live wire can be directly connected to the power source and can cause a fatal electric shock.
How does electromagnetic induction result in the generation of electricity in a power plant?
In power plants, turbines rotate a coil in a magnetic field, inducing an electromotive force due to electromagnetic induction. This leads to the generation of electricity.
What is static electricity, and how can it be produced?
Static electricity is produced when certain materials are rubbed together, causing electrons to transfer and resulting in one object gaining a surplus of electrons (negative charge) and the other losing electrons (positive charge).
Explain how a three-pin plug works and the function of each wire.
In a three-pin plug, the live wire carries the current to the appliance, the neutral wire carries it away, and the earth wire is a safety device that directs any leaked current into the earth.
What's the difference between conductors and insulators? Can you give two examples of each?
Conductors allow electric current to pass through them, while insulators do not. Examples of conductors include metals like copper and aluminum, while rubber and glass are examples of insulators.
What are electric fields and how do they influence charged particles?
Electric fields are regions around a charged particle in which an electric force is exerted on other charged particles. The direction of the field is the direction a positive charge would move if placed in the field.
Why do electrons move in a circuit?
Electrons move in a circuit because they are attracted to the positive terminal of the power source, such as a battery. This motion of electrons constitutes the electric current.
What is the difference between alternating current (AC) and direct current (DC)?
Direct current (DC) is a type of electrical current that flows in one direction, while alternating current (AC) changes direction periodically. Batteries provide DC, while the electricity supplied to homes is typically AC.
Why don't birds get electrocuted when they sit on power lines?
Birds don’t get electrocuted because they are not completing a circuit. They are only in contact with one line (and hence one voltage), not two different voltages, so there’s no potential difference across the bird, and no current flows through it.
What is the difference between voltage and current?
Voltage, measured in volts, is the electric potential difference between two points. It’s often thought of as the ‘push’ that drives charge carriers through a circuit. Current, measured in amperes (or amps), is the rate of flow of electric charge in a circuit.
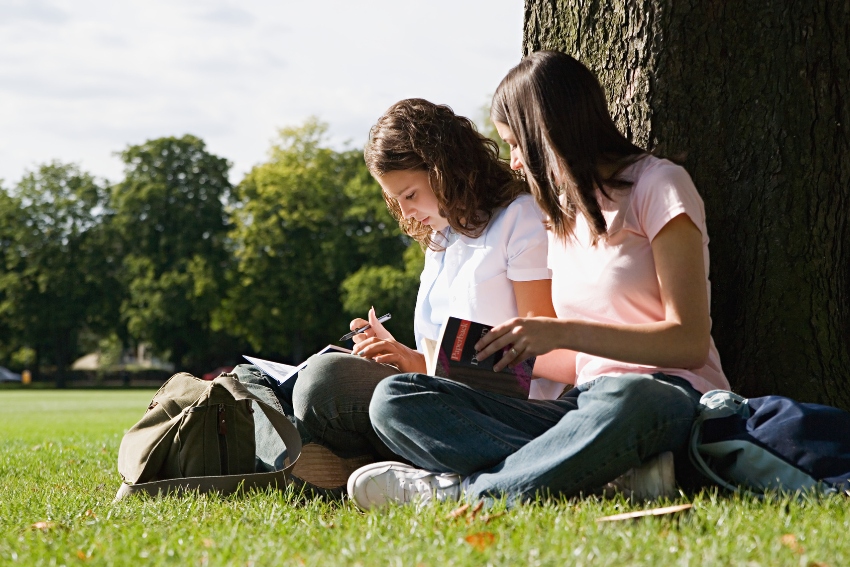