Key concepts to understand when it comes to atomic structure before we dive in deeper:
✏️ Atoms
Atoms are the smallest units of an element that retain its chemical properties. They consist of a central nucleus made up of protons and neutrons, surrounded by a cloud of electrons.
✏️ Nucleus
The nucleus is located at the center of an atom and contains positively charged protons and uncharged neutrons. Protons have a positive electrical charge, while neutrons are neutral or have no charge.
✏️ Electrons
Electrons are negatively charged particles that orbit around the nucleus in specific energy levels or shells. These energy levels are often represented as electron shells or electron clouds.
✏️ Atomic number
The atomic number of an atom is determined by the number of protons in its nucleus. It uniquely identifies each element on the periodic table. For example, hydrogen has an atomic number of 1, indicating that it has one proton in its nucleus.
✏️ Mass number
The mass number of an atom is the total number of protons and neutrons in the nucleus. It is represented by the symbol “A” and is used to identify different isotopes of an element. Isotopes are atoms of the same element that have different numbers of neutrons.
✏️ Electrons and energy levels
Electrons occupy specific energy levels or shells around the nucleus. The innermost shell can hold up to 2 electrons, while the subsequent shells can hold more. The electrons in the outermost shell, known as valence electrons, are involved in chemical bonding and interactions.
How do I determine the number of protons, neutrons, and electrons in an atom?
Protons, neutrons, and electrons are the essential characters in the atomic world, each with its unique role.
✏️ Protons
These positively charged particles are like the VIPs of the atom. They reside in the nucleus, which is the central core of the atom. The number of protons in an atom determines its atomic number, which identifies the element. For example, if an atom has 6 protons, it belongs to the element carbon, which has an atomic number of 6. So, to find the number of protons, simply look up the atomic number on the periodic table.
✏️ Neutrons
Neutrons are like the bodyguards of the nucleus. They have no charge, but they provide stability and balance by neutralizing the positive charge of protons. To find the number of neutrons, you need to know the mass number of the atom. The mass number is the sum of protons and neutrons. Subtract the atomic number (number of protons) from the mass number, and you’ll discover the number of neutrons hiding within the nucleus.
✏️ Electrons
Electrons are the energetic little particles that orbit around the nucleus. Think of them as the dancers at an atomic disco party! Electrons have a negative charge and are responsible for the chemistry and bonding behavior of atoms. The number of electrons in a neutral atom is equal to the number of protons. For example, if an atom has 8 protons, it will also have 8 electrons to balance the charges and maintain overall neutrality.
What is the significance of the atomic number and mass number?
Imagine you’re at a grand ball where each element is dressed up uniquely, and the atomic number and mass number hold the keys to understanding their true identities and characteristics.
✏️ Atomic Number
Picture the atomic number as a fancy entrance ticket to the ball. It’s like a special code assigned to each element, providing crucial information about its identity. The atomic number represents the number of protons in an atom’s nucleus. For example, hydrogen, the lightest element, has an atomic number of 1, indicating it has one proton. This atomic number serves as a unique identifier for elements on the periodic table, helping us distinguish one element from another. It’s like a name tag that instantly tells you which element is ready to mingle and make chemical connections.
✏️ Mass Number
Now let’s talk about the grandeur of the mass number. It’s like the sumptuous gown worn by an element, representing its total mass. The mass number reveals the combined number of protons and neutrons in an atom’s nucleus. Think of it as the element’s weight, indicating how heavy it is. However, since electrons are relatively lighter, their mass is not considered when calculating the mass number. By subtracting the atomic number from the mass number, we can find the number of neutrons, which adds to an atom’s overall mass. The mass number allows us to differentiate between different isotopes of the same element. So, just as different gowns can make elements of the same type look slightly different, isotopes have a subtle variation in mass due to the different number of neutrons they possess.
How do electrons occupy different energy levels or shells?
Imagine electrons as energetic little performers ready to dazzle on different stages called energy levels or electron shells.
✏️ Energy Levels
Think of energy levels as floors in an electron’s towering dance club. Each floor represents a specific amount of energy that electrons can have. The lowest energy level, also known as the ground state, is closest to the nucleus. As we move to higher floors, the energy of electrons increases. These energy levels are labeled as the first (closest to the nucleus), second, third, and so on.
✏️ Shell Capacity
Just like dance floors have a limited capacity, each energy level or electron shell has a specific maximum number of electrons it can hold. The first energy level can hold a maximum of 2 electrons, the second can hold up to 8 electrons, and the third level can accommodate a maximum of 18 electrons. It’s like a dance floor rule where the first floor can only hold two dancers, the second can hold eight, and the third can accommodate eighteen energetic performers.
✏️ Filling Order
Now, let’s talk about the electron filling order, which is like the choreography for these energetic dancers. Electrons follow a specific set of rules to fill the energy levels. The first energy level is filled before moving on to the second, and so on. Additionally, electrons tend to occupy lower energy levels before higher ones. This is known as the Aufbau principle, which ensures that the dance floors are filled in a systematic and orderly fashion.
✏️ Valence Electrons
The outermost energy level of an atom is particularly important and is known as the valence shell. The electrons in this shell are called valence electrons. Valence electrons determine an atom’s chemical behavior and its ability to form bonds with other atoms. These electrons are like the showstoppers, capturing attention and driving chemical reactions.
What are valence electrons, and why are they important?
Get ready to shine the spotlight on valence electrons, the captivating stars of the atomic show! Let’s unravel the mystery behind valence electrons and understand why they hold such significance.
✏️ Valence Electrons
Picture valence electrons as the glimmering jewels adorning the outermost energy level or shell of an atom. These are the electrons that are most involved in the atom’s interactions and bonding with other atoms. Valence electrons determine the chemical behavior and characteristics of an element.
✏️ Chemical Bonding
Valence electrons are crucial players in the formation of chemical bonds. Atoms strive to achieve a stable and harmonious state, often by gaining, losing, or sharing electrons. Valence electrons play a vital role in this dance of bonding. When two or more atoms come together, their valence electrons interact and form chemical bonds, connecting the atoms into molecules or compounds. The number and arrangement of valence electrons influence the types of bonds an atom can form and the overall structure of the resulting compound.
✏️ Reactivity
Valence electrons also dictate an atom’s reactivity. Elements with a few valence electrons tend to be highly reactive as they seek to either gain or lose electrons to achieve a stable electron configuration. These elements can easily participate in chemical reactions to achieve a more favorable electron arrangement. On the other hand, elements with full or stable configurations of valence electrons are typically less reactive.
✏️ Periodic Trends
The arrangement of valence electrons follows certain patterns across the periodic table. Elements in the same group or column often have similar valence electron configurations, leading to comparable chemical properties. Understanding the arrangement of valence electrons can help predict an element’s behavior, such as its tendency to gain or lose electrons.
What are isotopes, and how do they differ from each other?
Get ready to dive into the fascinating world of isotopes, where atoms come in different flavors! Let’s explore what isotopes are and how they differ from one another.
✏️ Isotopes
Think of isotopes as siblings from the same element family who share similar characteristics but have slight differences. Isotopes are atoms of the same element that have the same number of protons (which determines the element’s identity) but differ in the number of neutrons in their nuclei.
✏️ Mass Number
The mass number of an isotope represents the sum of protons and neutrons in its nucleus. Since isotopes have a different number of neutrons, their mass numbers vary. This means that isotopes of the same element have slightly different atomic masses. For example, carbon-12 and carbon-14 are both isotopes of carbon. Carbon-12 has 6 protons and 6 neutrons, giving it a mass number of 12. Carbon-14, on the other hand, has 6 protons and 8 neutrons, giving it a mass number of 14.
✏️ Properties and Stability
Although isotopes of the same element have different mass numbers, their chemical properties remain similar. This is because chemical properties primarily depend on the number of protons and electrons, which are the same for all isotopes of an element. However, the different number of neutrons can impact an isotope’s stability and nuclear properties. Some isotopes may be stable, while others can be radioactive and undergo radioactive decay over time.
✏️ Uses and Applications
Isotopes find numerous applications in various fields. For example, isotopes are used in radiometric dating to determine the age of artifacts and fossils. They are also employed in medical diagnostics and treatment, such as in PET scans and radiation therapy. Additionally, isotopes play a vital role in nuclear power generation and scientific research.
Test Yourself
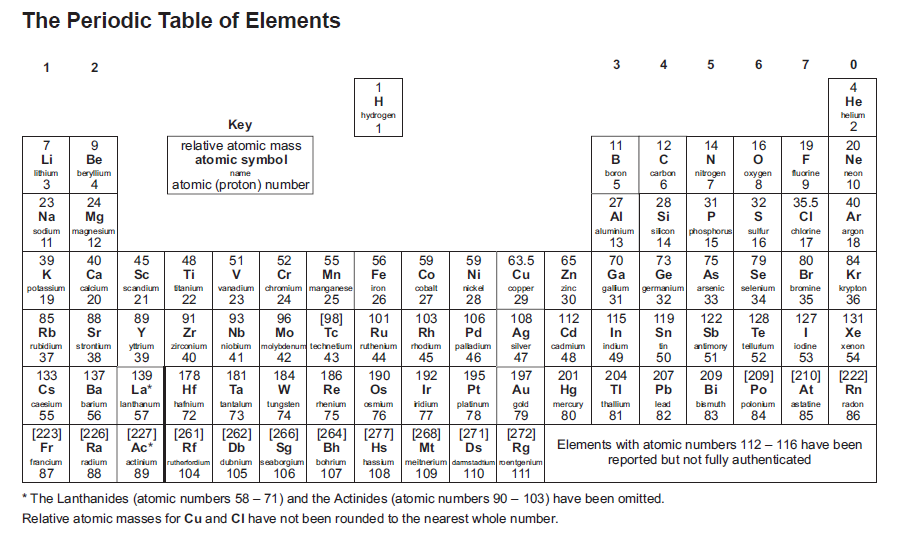
Question: Identify the atomic number, mass number, and number of neutrons for an atom with 16 protons and 18 neutrons.
Answer: Atomic number: 16, Mass number: 34, Number of neutrons: 18
Question: Draw the electron configuration for oxygen (atomic number 8) using the orbital notation (boxes and arrows) method.
Answer: Students should draw a diagram with two boxes in the first energy level (1s) and six boxes in the second energy level (2s and 2p orbitals) with arrows representing the electrons.
Question: Which element has the electron configuration 1s^2 2s^2 2p^6 3s^2 3p^5?
Answer: Chlorine (Cl)
Question: Calculate the number of valence electrons in an atom of phosphorus (atomic number 15).
Answer: Phosphorus has 5 valence electrons.
Question: How many electrons can the third energy level (n = 3) hold at maximum?
Answer: The third energy level can hold a maximum of 18 electrons.
Question: Name two isotopes of carbon, specifying their atomic numbers, mass numbers, and number of neutrons.
Answer: Carbon-12 (6 protons, 12 mass number, 6 neutrons) and Carbon-14 (6 protons, 14 mass number, 8 neutrons)
Question: Draw the Bohr model representation of an atom with 17 electrons.
Answer: Students should draw a nucleus with 17 protons and arrange the electrons in energy levels with 2, 8, 7 distribution (2 in the first level, 8 in the second level, and 7 in the third level).
Question: Which element has the electron configuration 1s^2 2s^2 2p^6 3s^2 3p^6 4s^1?
Answer: Potassium (K)
Question: Calculate the number of protons, neutrons, and electrons in an atom with mass number 23 and atomic number 11.
Answer: Protons: 11, Neutrons: 12 (mass number – atomic number), Electrons: 11 (for a neutral atom)
Question: Which element has 6 valence electrons and is in Group 16 (Group 6A) of the periodic table?
Answer: Oxygen (O)
Question: How many electrons are present in the third energy level (n = 3) of an atom with atomic number 13?
The third energy level can hold a maximum of 18 electrons, but in the case of aluminum (atomic number 13), it only has 3 electrons.
Question: Identify the element with the electron configuration 1s^2 2s^2 2p^6 3s^2 3p^6 4s^2 3d^10 4p^4.
Answer: The element is Selenium (Se).
Question: Draw the Lewis dot structure for nitrogen (atomic number 7) and determine the number of lone pairs and bonding pairs of electrons.
Answer: The Lewis dot structure of nitrogen shows a lone pair (two electrons) and three bonding pairs (six electrons).
Question: Which isotopes are used as fuel in nuclear reactors, and what are their symbols?
Answer: Uranium-235 (U-235) and Plutonium-239 (Pu-239) are commonly used isotopes as fuel in nuclear reactors.
Question: Calculate the average atomic mass of an element that has two isotopes: Isotope A with a mass of 25 amu (abundance of 70%) and Isotope B with a mass of 29 amu (abundance of 30%).
Answer: The average atomic mass can be calculated as follows: (25 amu * 0.70) + (29 amu * 0.30) = 26.5 amu
Additional Knowledge – Atomic Structure – GCSE Chemistry
Law of Conservation of Mass
The Law of Conservation of Mass simply means that in any chemical reaction, the total amount of mass or matter before the reaction is the same as the total amount of mass or matter after the reaction. This law suggests that atoms are neither created nor destroyed during a chemical reaction, but rather rearranged to form new substances. So, if you have a certain amount of stuff (atoms) at the beginning, you will end up with the same amount of stuff (atoms) at the end, just arranged differently. It’s like rearranging the furniture in a room – you don’t create or lose any furniture, you’re just moving it around. The Law of Conservation of Mass helps us understand that matter is conserved, and it plays a crucial role in understanding chemical reactions and balancing chemical equations.
Law of Definite Proportions
The Law of Definite Proportions, also known as the Law of Constant Composition, states that a chemical compound always contains the same elements in fixed proportions by mass. In simpler terms, it means that the ratio of the masses of the different elements in a compound is always the same.
For example, let’s consider water (H2O). The Law of Definite Proportions tells us that in every sample of water, the ratio of hydrogen (H) to oxygen (O) by mass will always be 2:16 or 1:8. This means that no matter how much water you have, the ratio of hydrogen to oxygen will always be the same.
This law highlights the consistent and predictable nature of compounds. It implies that the arrangement of atoms in a compound is fixed, and any variation in the amount of one element will result in a corresponding change in the amount of another element to maintain the same ratio.
Understanding the Law of Definite Proportions helps chemists determine the composition of compounds and provides a foundation for stoichiometry, which involves calculating the quantities of reactants and products in chemical reactions.
Law of Multiple Proportions
The Law of Multiple Proportions states that when two elements combine to form different compounds, the ratio of the masses of one element that combine with a fixed mass of the other element can be expressed in small whole numbers.
In simpler terms, it means that when two elements combine to form different compounds, the masses of one element that combine with a fixed mass of the other element will always have a simple ratio.
For example, let’s consider carbon (C) and oxygen (O). Carbon can combine with oxygen to form two different compounds: carbon monoxide (CO) and carbon dioxide (CO2). According to the Law of Multiple Proportions, the ratio of carbon to oxygen in carbon monoxide is 1:1, while in carbon dioxide, it is 1:2. The ratios are simple whole numbers.
This law suggests that elements can combine in different proportions to form distinct compounds with unique properties. The ratio of the masses reflects the arrangement of atoms in these compounds.
The Law of Multiple Proportions helps in understanding the concept of chemical formulae and the relationships between different compounds containing the same elements. It provides evidence for the discrete nature of matter and the existence of distinct compounds with specific ratios of elements.
Dalton’s Atomic Theory
Dalton’s Atomic Theory, proposed by John Dalton, is a fundamental theory that revolutionized our understanding of atoms and their role in chemical reactions. The theory consists of several key principles:
- All matter is composed of tiny, indivisible particles called atoms: According to Dalton, atoms are the fundamental building blocks of matter and cannot be further divided into smaller particles.
- Atoms of the same element are identical in mass and properties: Dalton suggested that all atoms of a particular element are identical in their mass, chemical properties, and behavior. This means that all atoms of oxygen, for example, have the same mass and exhibit similar chemical properties.
- Atoms of different elements have different properties: Dalton proposed that atoms of different elements vary in their mass and chemical properties. This difference in properties is what gives each element its unique characteristics.
- Chemical reactions involve the rearrangement of atoms: According to Dalton, chemical reactions occur when atoms rearrange to form new combinations. During a chemical reaction, atoms are neither created nor destroyed; they simply rearrange to form different compounds.
Dalton’s Atomic Theory laid the foundation for modern chemistry and significantly contributed to our understanding of atomic structure and the behavior of matter. While some of Dalton’s original ideas have been modified or expanded upon with more advanced scientific discoveries, his theory remains a fundamental concept in the study of atoms and chemical reactions.
Thomson’s Plum Pudding Model
Thomson’s Plum Pudding Model, proposed by J.J. Thomson, was an early atomic model that suggested the structure of an atom. It was proposed in the early 20th century before the discovery of the atomic nucleus.
According to Thomson’s model, an atom was envisioned as a sphere of positive charge with negatively charged electrons embedded within it, resembling plums in a pudding. The positive charge represented the overall positive charge of the atom, while the electrons were dispersed throughout the atom.
Thomson’s model was based on his experiments with cathode rays, which are streams of negatively charged particles (electrons) emitted from a negatively charged electrode called the cathode. He observed that these rays could be deflected by electric and magnetic fields, indicating the presence of negatively charged particles.
Thomson proposed that atoms were composed of a uniform positive charge distributed throughout the atom, and the negatively charged electrons were embedded within it. This model explained the existence of negatively charged particles within an atom and their ability to move.
However, the Plum Pudding Model was later replaced by Ernest Rutherford’s nuclear model, which provided evidence for the existence of a small, dense, positively charged nucleus at the center of the atom, with electrons orbiting around it. Rutherford’s experiments, such as the gold foil experiment, demonstrated that most of the mass and positive charge of an atom is concentrated in the nucleus, rather than being uniformly distributed throughout the atom.
While Thomson’s Plum Pudding Model was eventually superseded, it represented an important step in the development of atomic theory and contributed to our understanding of the presence of subatomic particles within atoms.
Rutherford’s Gold Foil Experimen
Rutherford’s Gold Foil Experiment, conducted by Ernest Rutherford in 1911, was a groundbreaking experiment that provided evidence for the existence of the atomic nucleus and led to a new understanding of atomic structure.
In this experiment, Rutherford and his colleagues directed a beam of alpha particles (positively charged particles) at a thin sheet of gold foil. They expected the alpha particles to pass through the gold foil with minimal deflection, based on the prevailing model at the time (Thomson’s Plum Pudding Model), which proposed that atoms were composed of a uniform positive charge with embedded electrons.
However, the results of the experiment were unexpected and revolutionary. While most of the alpha particles passed through the gold foil without any significant deflection, a small fraction of the particles were deflected at large angles, and a few even bounced straight back.
This observation led Rutherford to conclude that the deflections could only be explained if the atom contained a small, dense, and positively charged nucleus at its center. He proposed that most of the mass and positive charge of the atom were concentrated in this nucleus, while the negatively charged electrons orbited around it at a distance.
Rutherford’s Gold Foil Experiment challenged the prevailing atomic model of the time and provided strong evidence for the existence of a compact nucleus within the atom. It paved the way for the development of the nuclear model of the atom and revolutionized our understanding of atomic structure, leading to further discoveries and advancements in the field of nuclear physics.
Bohr’s Model of the Atom
Bohr’s Model of the Atom, proposed by Niels Bohr in 1913, is a model that describes the structure of atoms and the behavior of electrons within them. It built upon Rutherford’s nuclear model and introduced the concept of energy levels or electron shells.
According to Bohr’s model, electrons exist in specific energy levels or shells around the nucleus of an atom. Each energy level has a fixed amount of energy associated with it. The electrons occupy the lowest available energy level first before filling higher energy levels.
Bohr suggested that electrons could move between energy levels by absorbing or emitting energy in discrete amounts called quanta or photons. When an electron absorbs energy, it jumps to a higher energy level, and when it emits energy, it moves back to a lower energy level. The energy of the emitted or absorbed light is directly proportional to the energy difference between the energy levels.
Bohr’s model also introduced the concept of quantized orbits, implying that electrons could only occupy specific, well-defined orbits around the nucleus. These orbits, or electron shells, are represented by different quantum numbers (n=1, n=2, n=3, and so on), with each shell accommodating a maximum number of electrons.
The model successfully explained certain experimental observations, such as the discrete emission spectra observed for elements. It provided a framework for understanding the stability of atoms and the behavior of electrons in terms of energy levels.
While Bohr’s model had limitations and was later refined by quantum mechanics, it was an important step in the development of atomic theory. It contributed to our understanding of electron behavior and the quantised nature of energy levels in atoms.
Aufbau Principle
The Aufbau Principle, also known as the “building-up” principle, is a rule that governs the way electrons are arranged in atoms. It states that electrons fill the lowest energy levels or orbitals first before filling higher energy levels.
In simpler terms, the Aufbau Principle suggests that electrons occupy the lowest available energy level or orbital before moving to higher energy levels. It is similar to the idea that people enter a building starting from the ground floor and gradually move up to higher floors.
According to the Aufbau Principle, electrons fill the orbitals in a specific order based on their increasing energy levels. The energy levels are often labeled using the principle quantum number (n), where higher values of n correspond to higher energy levels.
For example, in the filling order of orbitals according to the Aufbau Principle, the 1s orbital is filled before the 2s orbital, and the 2s orbital is filled before the 2p orbital. The principle continues in this manner, with the 3s orbital filled before the 3p orbital, and so on.
The Aufbau Principle helps in understanding the electronic configuration of atoms, which refers to the distribution of electrons in various orbitals and energy levels. It provides a systematic approach for determining the order in which electrons occupy orbitals and allows us to predict the electron configurations of different elements in the periodic table.
Pauli Exclusion Principle
The Pauli Exclusion Principle, formulated by Wolfgang Pauli, is a fundamental principle in quantum mechanics that describes the behavior of electrons within an atom. It states that no two electrons in an atom can have the same set of quantum numbers.
In simpler terms, the Pauli Exclusion Principle asserts that within a given atom, no two electrons can occupy the exact same quantum state. This means that two electrons in the same atom must have different combinations of quantum numbers, which include properties such as energy, angular momentum, and spin.
To visualize this, imagine there are two seats available on a bus, and each seat has specific characteristics associated with it, like color and shape. According to the Pauli Exclusion Principle, two passengers (electrons) cannot occupy the same seat (quantum state) if they have the same combination of characteristics (quantum numbers). Each electron must have a unique combination of quantum numbers.
One important consequence of the Pauli Exclusion Principle is that it determines the maximum number of electrons that can occupy a given orbital. Each orbital can accommodate a maximum of two electrons, with opposite spins (spin up and spin down). This is why electron configurations in atoms follow specific patterns and why we observe the pairing of electrons in orbitals.
The Pauli Exclusion Principle helps explain various phenomena, such as the arrangement of electrons in atoms, the periodic table’s structure, and the stability of matter. It plays a crucial role in understanding the electronic structure of atoms and the behavior of electrons in chemical bonding and reactions.
Hund’s Rule
Hund’s Rule, named after Friedrich Hund, is a principle that governs the distribution of electrons in orbitals within a subshell. It states that when electrons occupy orbitals of the same energy level or subshell, they prefer to occupy separate orbitals with parallel spins before pairing up.
In simpler terms, Hund’s Rule suggests that electrons tend to occupy empty orbitals individually, with the same spin direction, before they start pairing up within a subshell.
To understand this principle, let’s consider the example of the p subshell, which has three orbitals (px, py, and pz) of the same energy level. According to Hund’s Rule, if we have three electrons to distribute among these orbitals, each electron will occupy a different orbital first, and they will all have the same spin. This means that each orbital will have one electron with the same spin before any orbital starts pairing up.
For instance, if we have three electrons in the p subshell, they will be distributed in the following way based on Hund’s Rule:
↑↓ (px) ↑↓ (py) ↑ (pz)
Here, the arrows represent electrons, and the upward arrow ↑ indicates an electron with one spin direction, while the downward arrow ↓ represents an electron with the opposite spin direction.
Hund’s Rule helps explain the electron configurations of atoms and the filling of orbitals in a way that maximizes electron-electron repulsion. By occupying separate orbitals with parallel spins, electrons can minimize their repulsive interactions, leading to a more stable arrangement.
Understanding Hund’s Rule is important in determining the electron configurations of atoms and predicting their chemical properties, as it influences the arrangement and behavior of electrons in bonding and chemical reactions.
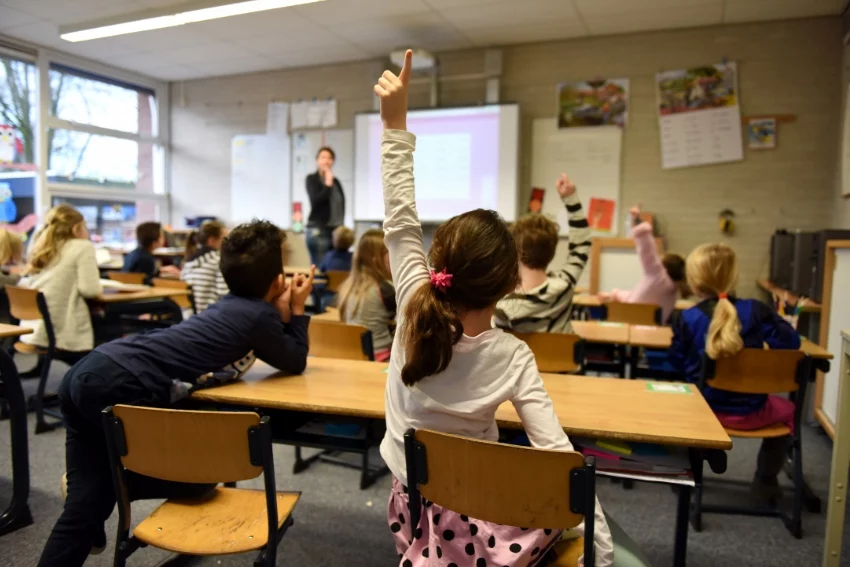