Table of Contents
- Cell Theory
- Theory of Evolution
- Mendelian Genetics
- DNA Structure and Replication
- Photosynthesis
- Respiration
- Homeostasis
- Osmosis and Diffusion
- Ecological principles
- Classification of living things
- Test Yourself
- Additional Knowledge – GCSE Biology – Organisation
- What is an organ system?
- What is the difference between an organ and a tissue?
- How does the digestive system work?
- What is the function of enzymes in the human body?
- What happens when enzymes are denatured?
- What are the main types of human blood cells and their functions?
- How does the circulatory system work?
Cell Theory
The Cell Theory is a fundamental concept in biology, and it’s about as essential to biology as the theory of gravity is to physics. So, let’s delve into it.
Imagine you’re constructing a building. You wouldn’t start with a whole wall or a floor – you’d start with bricks, right? Just like a building is made of individual bricks, all living organisms are made of individual building blocks called ‘cells’.
That’s the first part of the Cell Theory: all living things are made of cells. They can be single-celled, like bacteria and yeast, where the whole organism is just one cell. Or they can be multicellular, like humans, trees, and dogs, where the organism is made of many cells working together.
The second part of the theory states that the cell is the basic unit of life. This means that the cell is the smallest thing that can carry out all the functions of life, such as growth, metabolism, response to the environment, and reproduction. In our building analogy, it’s like saying each brick is a mini-building in itself with all the basic functions intact.
Lastly, the theory says that all cells come from pre-existing cells. Cells don’t just pop into existence; they are born from other cells. This happens when a cell divides into two, a process called cell division. It’s like if each brick could magically divide into two new bricks.
So in simple terms, Cell Theory tells us that: life’s building blocks are cells, cells have all the functions needed for life, and new cells are made when old cells divide. It’s one of the cornerstones that our understanding of biology is built upon!
Theory of Evolution
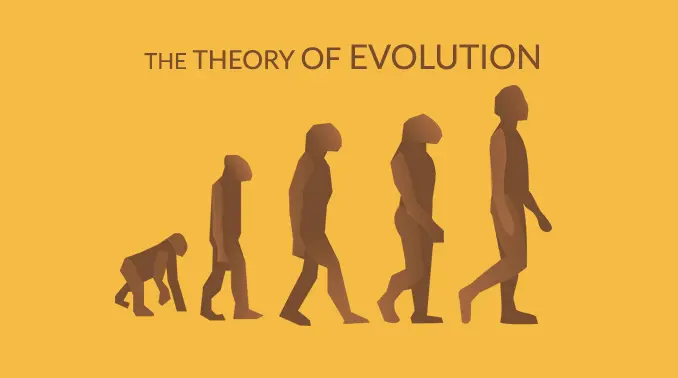
Imagine a group of people each trying to reach the top shelf of a cupboard to get a cookie. Only those tall enough can reach the cookies. Those who can reach the cookies get to eat, grow stronger, and eventually, when they have children, their kids are likely to be tall as well, since height is hereditary. Over time, the short people who couldn’t reach the cookies might become fewer because they’re not as healthy and strong. This means, in this scenario, being tall gives an advantage. By the way, don’t get us wrong, cookies are not healthy! Read about brain foods here.
This is a simplified way of looking at the Theory of Evolution, proposed by Charles Darwin. Here’s how it applies to the natural world:
Variation
Just like people in our example had different heights, individuals in a species have variations too. These variations or differences could be in color, size, speed, resistance to disease, and so on.
Struggle for Existence
Food and resources are limited. Species reproduce quickly and in large numbers, creating more offspring than the environment can support. This leads to a struggle for survival, just like everyone in our example couldn’t get the cookie.
Survival of the Fittest
Those individuals with variations that give them an advantage in their environment are more likely to survive and reproduce, like the tall people in our example. This is also known as natural selection.
Inheritance of Traits
The advantageous traits are likely to be passed on to the next generation. Over time, these traits become more common in the population.
Evolution of Species
Over many generations, the accumulation of these advantageous traits can lead to the development of a new species.
So, the Theory of Evolution explains how species change over time and why there’s so much diversity in the natural world. It’s based on the idea of ‘survival of the fittest’, where ‘fittest’ refers to the best suited to the current environment.
Mendelian Genetics
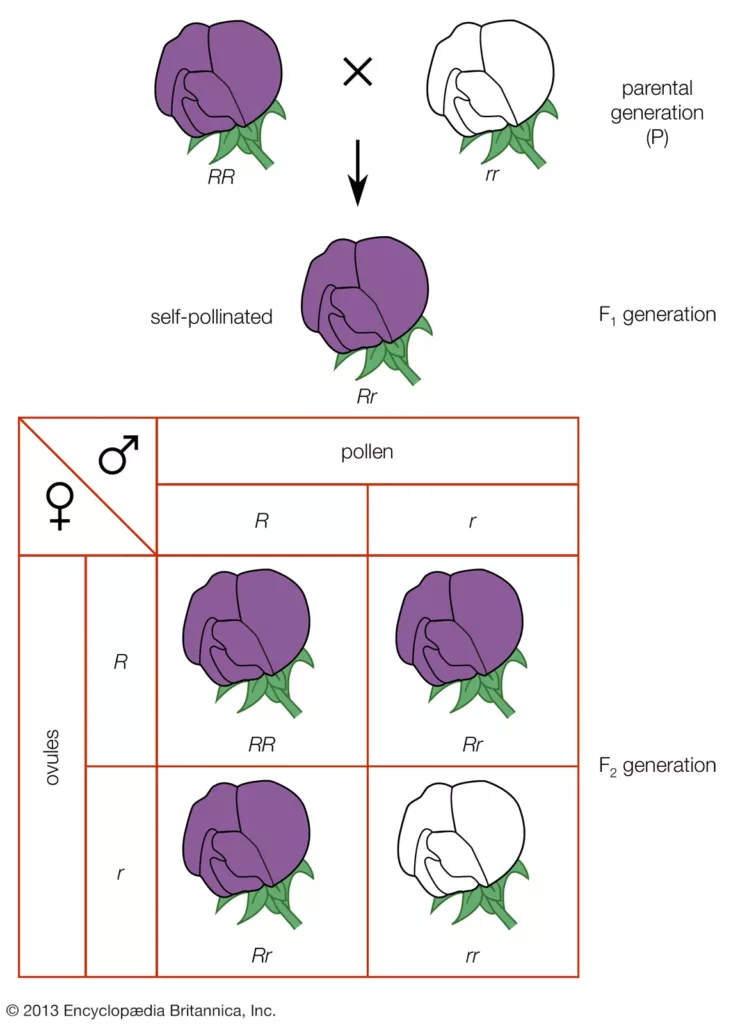
Let’s get into Mendelian Genetics, named after the scientist Gregor Mendel, who was a monk with a keen interest in pea plants. Here’s the simplified version:
Mendel’s garden was full of pea plants, and he noticed that they had different traits, like some had purple flowers, some had white; some were tall, some were short. He wondered how these traits were passed down through generations, so he started to experiment by crossing pea plants with different traits.
From these experiments, he formulated three key principles:
Principle of Dominance
Some traits seem to overpower others. Mendel noticed when he crossed a purple-flowered plant with a white-flowered one, all offspring in the first generation had purple flowers. Purple flower color was the dominant trait, and white was recessive.
Principle of Segregation
This principle says that the two copies of a gene separate from each other during the formation of gametes (sex cells). So, each gamete carries only one copy of each gene. This is why you have traits from both of your parents. Each of your parents’ gametes carried different genes, which combined to make you!
Principle of Independent Assortment
This principle states that different traits are inherited independently of each other. So, having purple flowers doesn’t mean a pea plant will necessarily be tall. The genes for flower color and height sort independently during gamete formation.
So, the basics of Mendelian genetics are that traits can be dominant or recessive, genes separate during the formation of gametes, and different traits are passed on independently of each other. Mendel’s principles laid the foundation for our understanding of how traits are passed from one generation to the next.
DNA Structure and Replication
DNA, short for deoxyribonucleic acid, is like a cookbook that contains the recipes, or ‘genes’, that make you uniquely you. It’s found in almost every cell of your body, inside a part called the nucleus.
- DNA Structure: DNA has a unique double helix structure, kind of like a twisted ladder. The sides of the ladder are made of sugar and phosphate molecules, and the rungs of the ladder are made of molecules called ‘bases’. There are four types of bases: Adenine (A), Thymine (T), Guanine (G), and Cytosine (C). These bases pair up in a specific way: A with T and G with C, creating base pairs that form the ‘rungs’ of the DNA ladder. It’s the order of these base pairs that forms the different ‘recipes’ or genes.
- DNA Replication: When a cell divides, it needs to make a copy of its DNA so each new cell has the complete cookbook. This is where DNA replication comes in. Here’s a simplified version of how it works:
- Unzipping: Imagine you’re unzipping a zip. That’s the first step of DNA replication. An enzyme called DNA helicase ‘unzips’ the DNA double helix into two single strands by breaking the bonds between the base pairs.
- Building New Strands: Each of these single strands serves as a template for a new strand. DNA polymerase is the enzyme that helps add new bases to the strand. It follows the rules of base pairing (A with T, and G with C) to match the bases on the original strand with new bases.
- Zipping Up: Once all the bases are matched up, you’ve got two identical copies of the DNA double helix, each one a mix of old and new strands. These are then ready to be packed into new cells during cell division.
And that’s a basic look at DNA structure and replication. It’s this incredibly intricate process that helps cells divide and allows life to continue and grow!
Photosynthesis
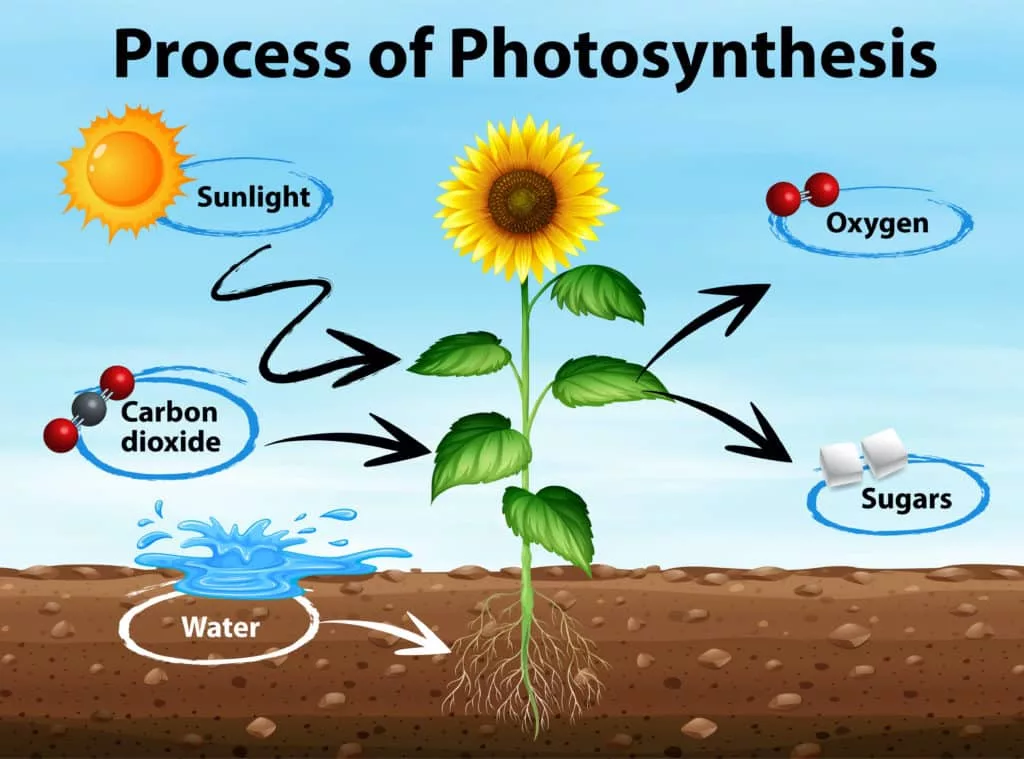
What is photosynthesis
Photosynthesis is the process that green plants, some bacteria, and algae use to convert light energy, usually from the sun, into chemical energy. They store this energy in the form of glucose (a type of sugar), which can be used later. It’s pretty amazing when you think about it – plants can capture and store sunlight!
Where does photosynthesis happen?
Photosynthesis takes place in a part of the plant cell called the chloroplast. Chloroplasts contain a green pigment called chlorophyll, which captures the light energy.
How does photosynthesis work?
Photosynthesis can be broken down into two main stages: the light-dependent reactions and the light-independent reactions (also known as the Calvin Cycle).
Light-dependent reactions
This stage happens when the plant is exposed to light. The chlorophyll in the chloroplasts absorbs light energy and uses it to split water molecules (which the plant has absorbed from the soil through its roots) into hydrogen and oxygen. The oxygen is released as a waste product (luckily for us, because we breathe it!).
Calvin Cycle (Light-independent reactions)
This stage doesn’t need direct light, but it does need the products of the light-dependent reactions. During the Calvin Cycle, the plant takes in carbon dioxide from the air and uses the energy from the light-dependent reactions to convert the carbon dioxide and hydrogen into glucose.
So in a nutshell, photosynthesis is the process where plants take in water, carbon dioxide, and light energy, and transform them into glucose and oxygen. This process is vital not just for the plants themselves, but for life on Earth as a whole, because it produces oxygen and contributes to the cycle of carbon dioxide.
Respiration
Respiration is the process used by all cells, in all living organisms, to produce energy from glucose. It’s kind of like burning fuel in a car engine, but instead of fuel, cells ‘burn’ glucose. This process happens in tiny structures in the cell called mitochondria.
Respiration can happen in two ways, depending on the availability of oxygen: aerobic respiration (requires oxygen) and anaerobic respiration (doesn’t require oxygen).
- Aerobic Respiration: This is the process most of us think of when we hear “respiration”. It’s the process of breaking down glucose with the help of oxygen to produce energy. The equation for this is: Glucose + Oxygen → Carbon dioxide + Water + Energy This process provides a lot of energy and is the preferred method of respiration for most cells, especially in animals and plants.
- Anaerobic Respiration: This happens when there’s not enough oxygen available. Since the cell still needs energy to survive, it uses this alternative method. However, anaerobic respiration doesn’t produce as much energy as aerobic respiration. In humans and many other animals, the equation for anaerobic respiration is: Glucose → Lactic acid + Energy In yeast cells (and in human muscle cells under intense activity), the equation is: Glucose → Ethanol + Carbon dioxide + Energy
To summarize, respiration is the process of producing energy. Whether oxygen is present or not determines if the respiration is aerobic or anaerobic. This process is essential because it provides the energy that cells need to perform all their functions.
Homeostasis
Homeostasis is like your body’s personal thermostat and regulation system. It’s all about maintaining a stable internal environment, even when the outside environment changes.
Think about it this way. Your house needs to be at a comfortable temperature, not too hot and not too cold. So, when it gets too hot, the air conditioning kicks in to cool the place down. If it gets too cold, the heater starts to warm things up. That’s pretty much how homeostasis works.
Here are some examples of how homeostasis operates in your body:
- Body Temperature: Your body needs to keep a constant temperature around 37°C (98.6°F) to function effectively. If you’re too cold, you’ll shiver to generate heat. If you’re too hot, you’ll sweat to lose heat and cool down.
- Blood Glucose Levels: Your body needs to keep the right amount of glucose (sugar) in your blood. Too much or too little can cause problems. When you eat, your blood glucose levels rise. In response, your pancreas produces insulin to help your cells absorb the glucose. If you haven’t eaten for a while, your blood glucose levels drop, and your pancreas produces glucagon to make your liver release stored glucose.
- Water Balance: Your body needs to balance the amount of water you take in (through drinking and eating) with the amount you lose (through sweating, breathing, and urinating). If you’re dehydrated, your brain will make you feel thirsty so you drink more water.
- Blood Pressure: Your body needs to maintain a steady blood pressure. If it’s too high or too low, your heart rate will change, or your blood vessels will adjust their width to bring it back to normal.
So, homeostasis is about balance. It’s your body’s way of making sure everything is just right for your cells to work properly. It involves a lot of different systems working together and responding to changes, all to keep you running smoothly!
Osmosis and Diffusion
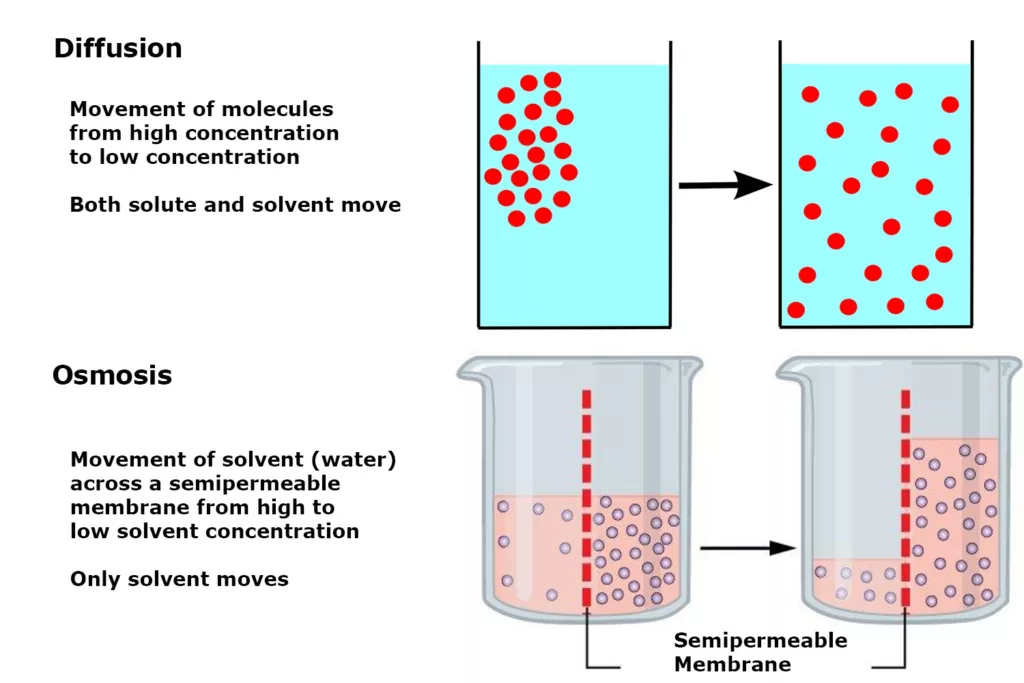
Diffusion
Imagine being in a room when someone sprays some perfume. At first, you might only smell it if you’re nearby. But give it a few minutes, and you’ll start to smell it no matter where you are in the room. That’s diffusion in action!
Diffusion is the movement of molecules from an area of higher concentration to an area of lower concentration. It’s like the molecules want to spread out and evenly fill the space they’re in. They continue to move and mix until they are evenly distributed. So, in the case of the perfume, the scent molecules spread out from the high concentration near the perfume bottle to the lower concentration in other parts of the room.
Osmosis
Now, let’s think about a raisin soaking in a cup of water. After a while, the raisin swells up because it’s absorbed water. This happens because of osmosis.
Osmosis is a special type of diffusion. It’s the movement of water from an area of higher water concentration (where there’s more water molecules) to an area of lower water concentration. This movement happens across a semi-permeable membrane, which is a barrier that only allows certain things to pass through. In the case of the raisin, the skin acts as the semi-permeable membrane.
So, to sum up: diffusion is the process of molecules spreading out from where there’s a lot of them to where there’s fewer. Osmosis is a similar concept, but it’s specifically about the movement of water through a semi-permeable membrane.
Ecological principles
- Food Chains and Food Webs: Ecological relationships are often depicted through food chains and food webs. A food chain shows the flow of energy from one organism to another in a linear sequence. For example, grass is eaten by a grasshopper, which is then eaten by a frog, which is eventually consumed by a snake. A food web, on the other hand, represents a network of interconnected food chains, showing the multiple feeding relationships within an ecosystem.
- Energy Flow: Energy flows through ecosystems in a unidirectional manner. It begins with producers (usually plants) that capture sunlight and convert it into chemical energy through photosynthesis. This energy is then transferred to herbivores (primary consumers), which are subsequently consumed by carnivores (secondary and tertiary consumers). Energy is eventually lost as heat at each trophic level, limiting the length of food chains.
- Nutrient Cycling: Nutrients, such as carbon, nitrogen, and phosphorus, move through ecosystems in a cyclic manner. Decomposers, such as bacteria and fungi, break down dead organic matter and return nutrients back to the soil, where they can be taken up by plants again. This cycling ensures a continuous supply of nutrients for living organisms.
- Population Dynamics: Populations of organisms are constantly changing in size and distribution. Factors such as birth rates, death rates, immigration, and emigration influence population dynamics. The interplay between these factors determines whether a population increases, decreases, or remains stable over time.
- Biodiversity: Biodiversity refers to the variety of different species, genetic variation within species, and the variety of ecosystems within a given area. Biodiversity is important for the stability and resilience of ecosystems. It supports ecosystem services like pollination, nutrient cycling, and regulation of pests and diseases.
- Habitat Destruction and Conservation: Human activities, such as deforestation and habitat destruction, can have a significant impact on ecosystems and biodiversity. Conservation efforts aim to protect and restore habitats, preserve endangered species, and maintain ecosystem health for the benefit of present and future generations.
Understanding these ecological principles is crucial for comprehending the interconnectedness and dynamics of the natural world and how human actions can influence ecosystems.
Classification of living things
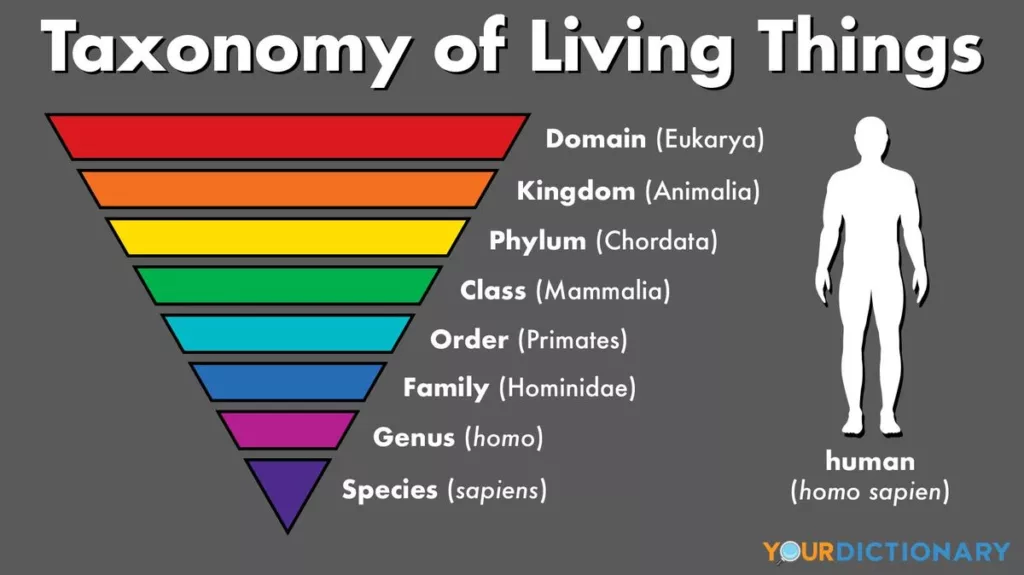
Living organisms are classified into a hierarchical system called taxonomy. This system helps organize and categorise the incredible diversity of life on Earth. The primary levels of classification, from broadest to most specific, are as follows:
- Domain: The highest level of classification, there are three domains: Archaea, Bacteria, and Eukarya. Archaea and Bacteria are composed of single-celled microorganisms, while Eukarya consists of organisms with more complex cell structures, including animals, plants, fungi, and protists.
- Kingdom: Each domain is further divided into kingdoms. For example, in the Eukarya domain, there are commonly recognized kingdoms such as Animalia (animals), Plantae (plants), Fungi (fungi), Protista (single-celled eukaryotes), and more.
- Phylum (plural: Phyla): Within each kingdom, organisms are grouped into phyla based on shared characteristics. For instance, in the Animalia kingdom, there are phyla such as Chordata (animals with a backbone) and Arthropoda (insects, spiders, and crustaceans).
- Class: Phyla are further divided into classes based on additional shared characteristics. In the class Mammalia (mammals), for example, organisms share features like mammary glands and hair or fur.
- Order: Classes are further divided into orders. In the class Mammalia, there are orders like Primates (primates including humans) and Carnivora (carnivorous mammals).
- Family: Orders are divided into families. For instance, within the order Primates, there is the family Hominidae, which includes humans and their closest relatives.
- Genus (plural: Genera): Families are then divided into genera. The genus Homo, for example, includes modern humans (Homo sapiens) and extinct human species like Homo neanderthalensis (Neanderthals).
- Species: Finally, the genus is divided into species. A species refers to a group of organisms that can interbreed and produce fertile offspring. For instance, Homo sapiens refers specifically to modern humans.
This hierarchical classification system helps scientists organize and understand the relationships between different organisms. It allows us to group similar organisms together while highlighting their unique characteristics and evolutionary history.
Test Yourself
What is the role of the pancreas in the digestive system?
The pancreas produces enzymes that help break down carbohydrates, proteins, and fats in the small intestine.
Why are enzymes important in biological systems?
Enzymes are important because they speed up chemical reactions in biological systems, making metabolism efficient.
What happens in the small intestine during digestion?
In the small intestine, digested food is absorbed into the bloodstream. This is where nutrients from the food enter the body.
What is the difference between arteries and veins in terms of their function and structure?
Arteries carry oxygen-rich blood away from the heart and have thick, muscular walls to withstand high pressure. Veins carry oxygen-poor blood back to the heart and have thinner walls and valves to prevent backflow.
How does the heart contribute to the circulatory system?
The heart is the pump that keeps the circulatory system working. It contracts to push blood throughout the body.
Why is a high fever dangerous in terms of enzyme function?
High fever is dangerous because it can denature enzymes. Enzymes work best at specific temperatures, and if the body temperature gets too high, it can change their shape, making them unable to perform their functions.
What is peristalsis and where does it occur?
Peristalsis is the series of wave-like muscle contractions that move food through the digestive tract. It occurs in the esophagus, stomach, and intestines.
What is the role of hemoglobin in red blood cells?
Hemoglobin in red blood cells binds to oxygen and carries it from the lungs to the rest of the body.
Which organ system is responsible for removing waste products from the bloodstream?
The excretory system, particularly the kidneys, is responsible for removing waste products from the bloodstream.
How does the structure of the stomach suit its function in digestion?
The stomach has a muscular wall that allows it to churn and mix food, breaking it down into a liquid. It also produces acid and enzymes that help break down food.
Additional Knowledge – GCSE Biology – Organisation
What is an organ system?
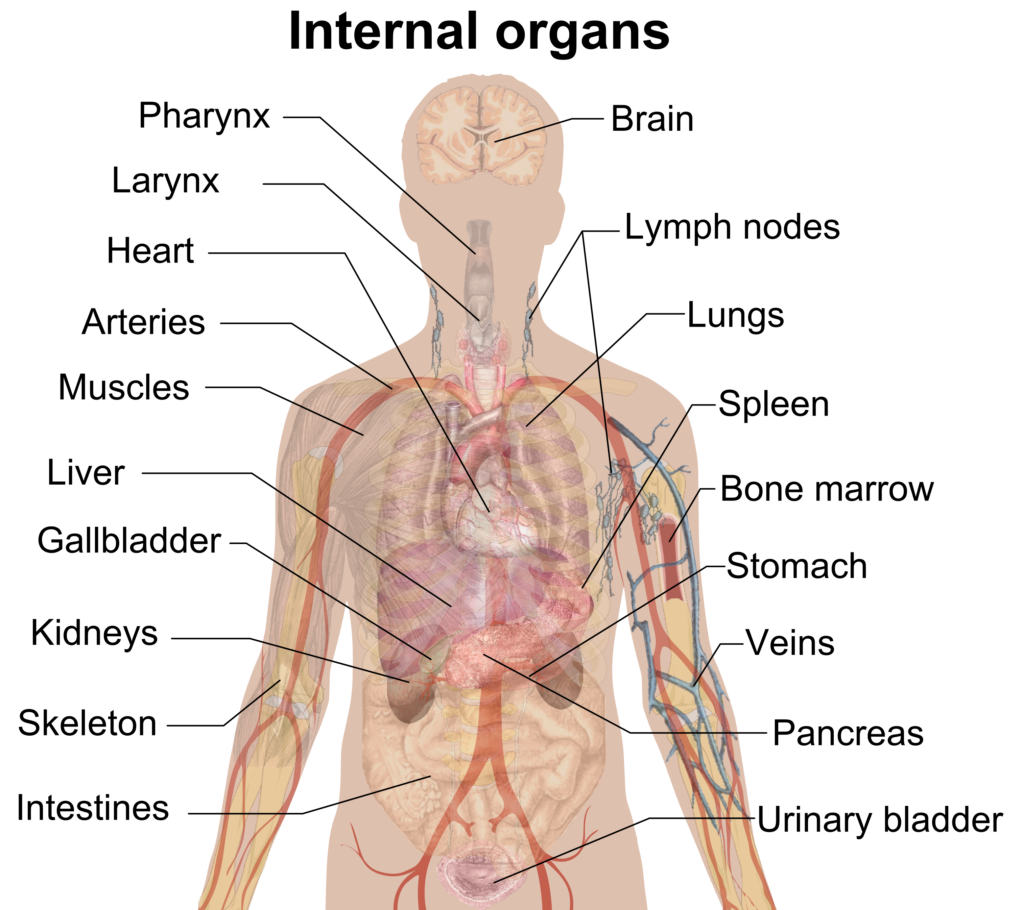
So, think about an organ system like a football team. It’s not just one player scoring all the goals; it’s a coordinated effort with different players performing specific tasks, right? They all play together to win the game.
In the same way, an organ system is a group of organs, each with its own role, working together to perform a major function for the body. These organs don’t function in isolation; they coordinate their actions and work as a unified team to help your body thrive.
Take the digestive system as an example. This ‘team’ includes several ‘players’ – the stomach, liver, pancreas, and intestines. They all work together to achieve the ‘goal’ of breaking down the food you eat into smaller molecules that your body can use for energy, growth, and cell repair.
The stomach starts the process by using its acid and enzymes to break down the food into a mushy substance. The liver produces bile to help dissolve fats, while the pancreas produces enzymes to help further break down the food. Then, the small intestine absorbs these smaller molecules into the bloodstream. Any leftover materials are passed to the large intestine, which removes them from the body.
So, organ systems are like these highly coordinated teams, each organ playing its specific role, but all working together towards a common goal – keeping your body functioning properly. This makes organ systems both fascinating and vital to understanding how our bodies work. Happy studying!
What is the difference between an organ and a tissue?
Think of building a house. When you start, you don’t immediately have a fully constructed house, right? You begin with bricks, assemble them into walls, and then put the walls together to build the house. Similarly, in the body, cells are like the ‘bricks’ and they group together to form ‘walls’ or tissues. And just like how we combine multiple walls to form a complete house, multiple tissues work together to form an organ.
A tissue is a collection of similar cells working together to perform a specific function. Let’s consider muscle tissue as an example – it’s made up of muscle cells which all contract to allow movement. You can think of tissue as the team players all playing the same position.
An organ, however, is like the whole team. It’s made up of different kinds of tissues all structured together to perform more complex tasks. Take the heart, for example. The heart isn’t just made up of one type of tissue. It’s primarily composed of muscle tissue (for pumping blood), but it also has connective tissue (for structure) and nervous tissue (for transmitting signals).
So, to sum it up, cells of the same type join forces to create tissues, then different types of tissues team up to form organs. Understanding this helps us appreciate the amazing teamwork happening in our bodies every moment of every day!
How does the digestive system work?
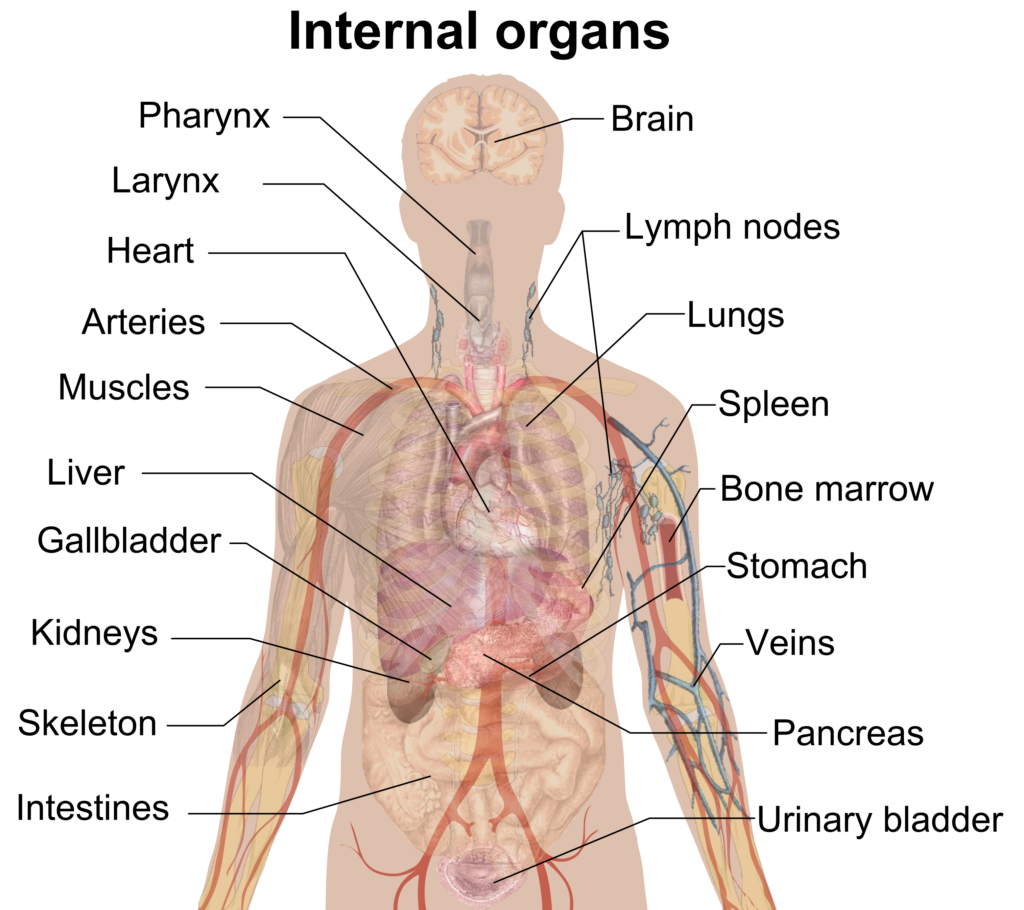
Picture your body as a well-oiled machine, with your digestive system acting like a top-notch fuel processing plant. Its job is to take in food (fuel), break it down into usable parts, and distribute these parts to where they’re needed. Any waste that can’t be used is then properly disposed of.
- Mouth: The process starts right when you take your first bite. Your teeth begin breaking down the food into smaller pieces, and your saliva adds enzymes that start to break down the food chemically. This mix is then formed into a small ball called a bolus, ready to travel down to the stomach.
- Esophagus: The bolus travels down this tube-like structure through a process called peristalsis, which is a fancy way of saying “muscle contractions”. It’s like a food slide that connects your mouth to your stomach.
- Stomach: Once the food arrives in the stomach, it’s greeted by stomach acids and more digestive enzymes. This potent mixture further breaks down the food, turning it into a soupy substance known as chyme.
- Small intestine: This is where the magic really happens. The chyme moves into the small intestine, where it’s broken down even more by enzymes produced by the liver and pancreas. The walls of the small intestine absorb the nutrients, which are tiny enough to pass into the bloodstream and be transported to the rest of the body. It’s like a delivery service that takes the nutrients to wherever they’re needed.
- Large intestine (colon): What’s left – mostly water and indigestible fibre – moves into the large intestine. Here, most of the remaining water is absorbed back into the body, and the undigested parts are formed into solid waste.
- Rectum and Anus: The solid waste, or feces, is stored in the rectum until it’s ready to be expelled from the body through the anus. This is the final stage of digestion, the point where the waste materials exit the system.
So, the digestive system is like your body’s personal fuel processing plant, converting food into the energy and nutrients your body needs, and efficiently dealing with the waste. This is how the human machine keeps running!
What is the function of enzymes in the human body?
Enzymes are like the unsung heroes of our bodies – always working behind the scenes to make sure everything runs smoothly. They are biological catalysts, and without them, chemical reactions in our body would be too slow to sustain life.
Here’s a cool way to imagine enzymes: think of them as incredibly skilled locksmiths. In any chemical reaction in your body, you have certain molecules, called substrates, that need to transform or combine in a specific way. But to do that, they need to fit together perfectly – like a key in a lock. Here’s where our enzyme locksmith comes into play!
Every enzyme has a unique shape that fits perfectly with its substrate (the ‘key’). This special area is called the active site. When the substrate enters the active site, the enzyme speeds up the reaction – it helps the ‘key’ turn, unlocking the reaction that transforms or combines the molecules. After the reaction, the enzyme remains unchanged, ready to catalyse another reaction. This process allows the body to carry out countless reactions quickly and efficiently.
Enzymes are everywhere in your body, playing crucial roles in many processes like digestion, where they help break down food into smaller molecules, and in DNA replication, where they help copy the DNA when cells divide.
So, enzymes might be invisible to us, but their actions are vital for our life. They are like master locksmiths that help keep the grand machinery of our bodies in motion!
What happens when enzymes are denatured?
When it comes to enzymes, their shape is everything.
Think of an enzyme like a master key. Each key (enzyme) has a unique shape that fits perfectly into a specific lock (the substrate). This ‘lock and key’ fit is what allows the enzyme to do its job in catalysing reactions.
Now, what if our key (enzyme) somehow gets bent or warped? It’s not going to fit into its lock (substrate) anymore, right? That’s essentially what happens when an enzyme gets denatured.
Enzyme denaturation is the process where an enzyme loses its shape, and this is usually due to changes in temperature or pH. Just like a key no longer working if it’s bent out of shape, once an enzyme is denatured, it can’t fit with its substrate and thus can’t perform its job of catalysing reactions.
It’s important to note that denaturation is often irreversible. So, once an enzyme has been denatured, it usually can’t be returned to its original shape. This can disrupt the normal functioning of the body, which is why it’s so important for the conditions in our bodies to stay within a certain range.
So, enzymes being denatured is kind of like a locksmith’s worst nightmare – the keys lose their shapes and can’t open their locks, disrupting the crucial chemical reactions they were supposed to help with.
What are the main types of human blood cells and their functions?
Absolutely, let’s talk about the main types of human blood cells:
- Red Blood Cells (RBCs) or Erythrocytes: These are the super transporters of the body. Imagine them as tiny, round delivery vans packed with a special molecule called hemoglobin. This molecule has a unique talent – it can pick up oxygen from the lungs and deliver it to all parts of the body. But RBCs aren’t one-trick ponies – after delivering oxygen, they collect carbon dioxide (a waste product) and bring it back to the lungs to be exhaled out of the body. So, they are constantly making these important deliveries, helping every part of the body get the oxygen it needs while getting rid of unwanted carbon dioxide.
- White Blood Cells (WBCs) or Leukocytes: These are the body’s defence force – think of them as the body’s security guards or soldiers. Their job is to protect the body against harmful invaders like bacteria, viruses, and other pathogens. They can detect these invaders and launch attacks to destroy them. There are different types of WBCs, each with its unique way of protecting the body, but they all work together to keep us healthy.
- Platelets or Thrombocytes: These are like the body’s emergency repair crew. When you get a cut or wound, they rush to the site and stick together to form a clot – like a bandage over the wound. This clot helps stop the bleeding and begins the healing process. Without platelets, even a small cut could potentially cause a lot of blood loss.
So, these are the three main types of blood cells: red blood cells delivering oxygen and removing carbon dioxide, white blood cells defending against harmful invaders, and platelets repairing damages. Together, they form an incredible team that keeps our bodies functioning, protected, and healed.
How does the circulatory system work?
Certainly! Let’s explore the circulatory system, which is your body’s very own delivery service, highway system, and waste removal service all in one:
- Delivery Service: The circulatory system transports vital substances to where they’re needed. Imagine red blood cells as tiny delivery trucks loaded with oxygen from the lungs. They travel through a vast network of blood vessels, delivering oxygen to cells all around the body. Once they’ve delivered their oxygen, they pick up carbon dioxide – a waste product – to be transported back to the lungs.
- Highway System: It’s not just oxygen and carbon dioxide that the circulatory system transports. It also carries nutrients from the food you’ve digested to the cells that need them, hormones from glands to target cells, and white blood cells and antibodies to areas where infection is taking place.
The circulatory system is made up of three key parts:
- Heart: This is like the central hub or command center. The heart pumps the blood, sending it on its journey around the body. It’s a powerful muscle that never takes a break, and it has four chambers to help it do its job: two atria on the top and two ventricles on the bottom. The heart’s pumping action is what creates our pulse.
- Blood Vessels: These are the actual ‘roads’ of our body. Arteries carry blood away from the heart, while veins return it. Capillaries are tiny vessels where the exchange of oxygen, nutrients, and waste occurs with the body’s cells.
- Blood: This is the transport medium, consisting of red blood cells (carry oxygen and carbon dioxide), white blood cells (fight infection), platelets (help clotting), and plasma (a fluid that carries nutrients, hormones, and waste).
- Waste Removal Service: Once the red blood cells have unloaded their oxygen, they pick up carbon dioxide – a waste product – from the cells. The blood then transports this carbon dioxide back to the lungs, where it’s exhaled out of the body.
So, your circulatory system is a sophisticated transportation network, always ensuring that every cell in your body gets what it needs to function and grow, and that waste products are efficiently removed. It’s one of the key reasons you’re alive and well!
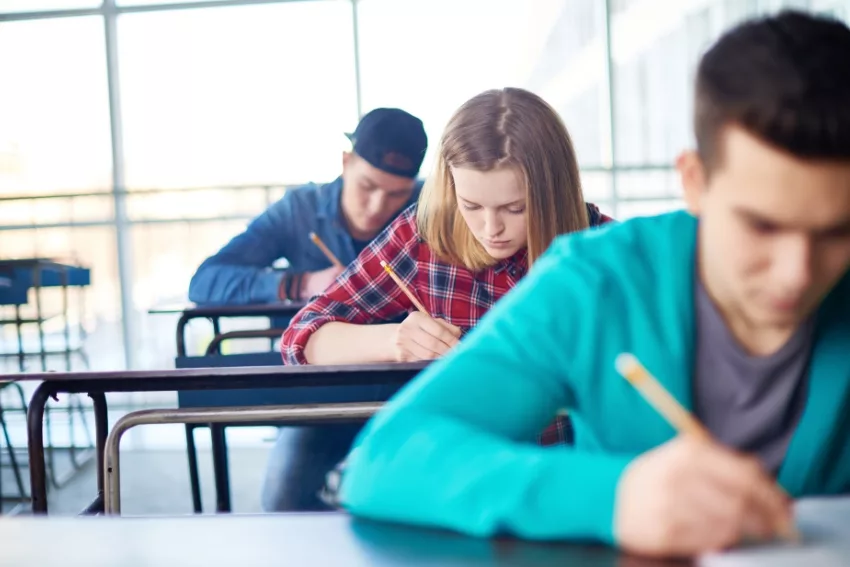