What is a Cell?
A cell is like a miniature, super complex factory, running 24/7, doing all sorts of amazing things that keep you alive and functioning. If you’ve ever seen how busy factories are – with lots of machines and people working to produce something – then you’ll have a good idea of what’s going on inside each of your cells.
In this factory, the boss is the nucleus. It contains DNA, which is basically the blueprint or instruction manual for everything the cell needs to do. When the factory needs to create something, the nucleus sends out specific instructions.
Think of mitochondria as the power generators. They’re like the batteries or the solar panels of the cell, converting nutrients into energy so that the cell can run its operations.
And it’s not just about building stuff. Cells also have to deal with waste. Lysosomes are like the cell’s recycling center. They break down waste materials and cellular debris.
There are other parts too, like ribosomes (the protein factories), the endoplasmic reticulum (the assembly line), and the Golgi apparatus (the packaging and shipping department), among others. Each cell is bustling with activity, just like a factory!
So next time you think of a cell, imagine it as this incredibly busy, super efficient factory, producing vital goods, managing waste, and powering all of its operations. Cells are the tiniest units of life, but without them, life as we know it would simply cease to exist.
What’s the Difference Between a Plant Cell and an Animal Cell?
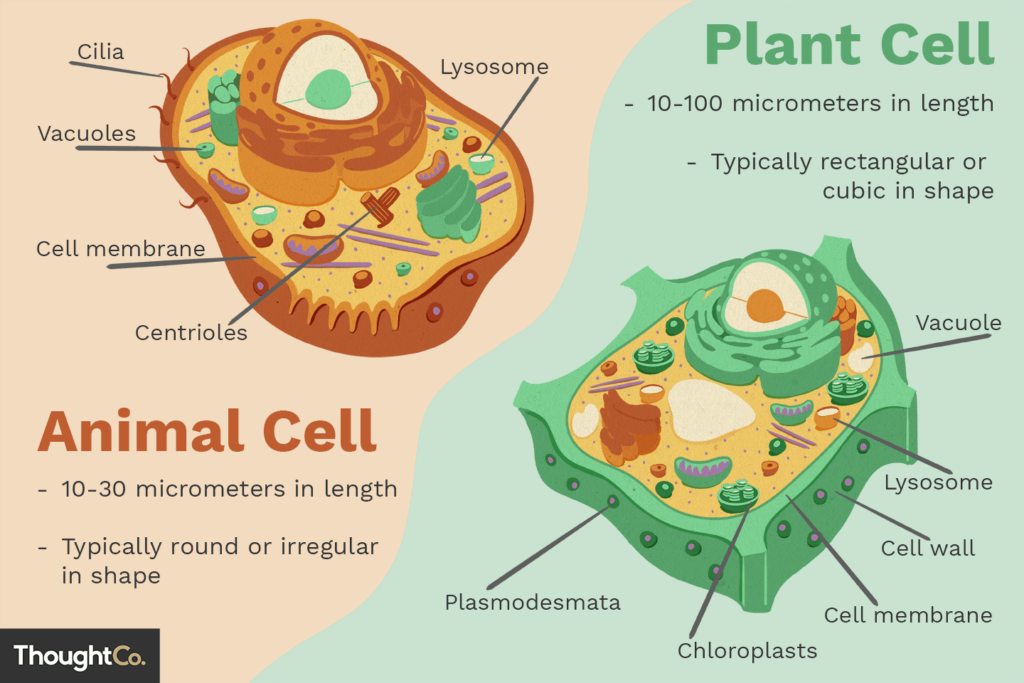
Think about the differences between a house and an apartment. They both provide shelter, have rooms, and utilities, but there are some key features that set them apart, right? It’s the same with plant cells and animal cells. They have a lot in common, but there are some important differences too.
Both types of cells have a nucleus (like the main control room), mitochondria (the power generators), and other basic cell machinery. But plant cells have three major features that animal cells don’t.
1. Cell Wall: Plant cells have a tough outer layer called the cell wall, kind of like the bricks of a house. It gives the cell strength and structure, and helps to resist pressure from the inside and outside. Animal cells, on the other hand, just have a flexible cell membrane, more like a tent than a solid brick house.
2. Chloroplasts: These are like little solar panels inside the plant cell that capture sunlight and convert it into energy through a process called photosynthesis. It’s kind of like having your own renewable energy source in your backyard. Animal cells don’t have this feature; they have to get their energy from food.
3. Central Vacuole: Plant cells have a large central vacuole that stores water and nutrients, kind of like a huge water tank or pantry. This helps the plant survive when resources are scarce. Animal cells do have vacuoles, but they’re much smaller and have different functions.
So, just like a house and an apartment have their own unique features, so do plant and animal cells. They’re both cells, but their differences are crucial for the survival of the organisms they belong to.
What are Eukaryotic and Prokaryotic Cells?
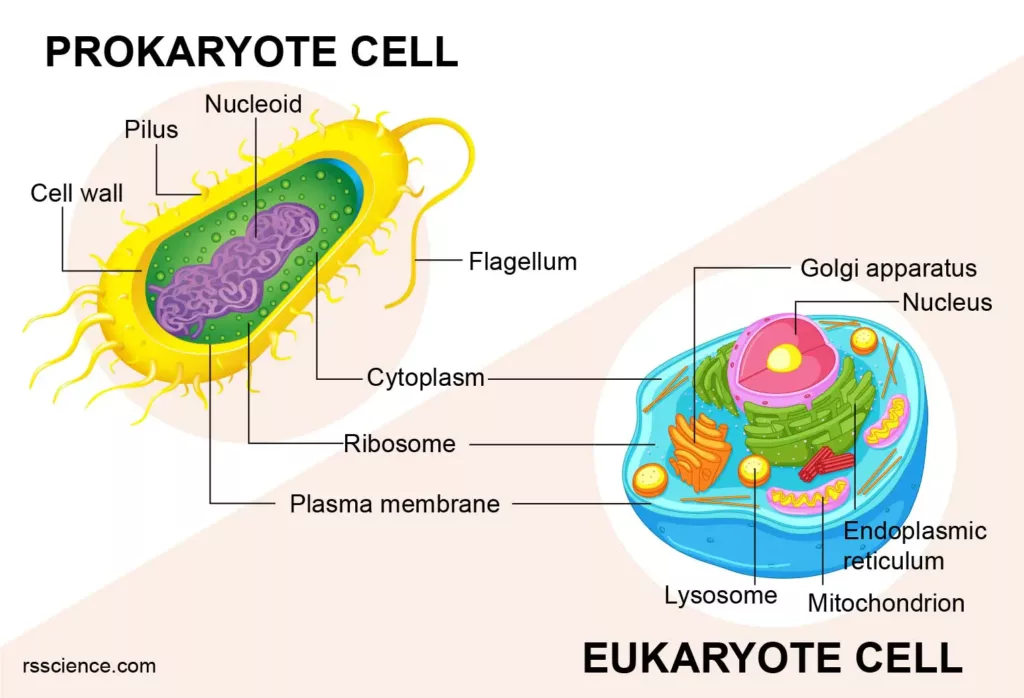
Imagine two types of houses: one is a large mansion with lots of rooms for different purposes, and the other one is a simple, open-plan studio apartment. Now, if these houses were cells, the mansion would be a eukaryotic cell, and the studio apartment would be a prokaryotic cell.
Eukaryotic Cells (The Mansion): These cells are like mansions with lots of specialised rooms, or ‘organelles.’ Each organelle has a specific function, like how a kitchen is for cooking, and a bedroom is for sleeping. The most important room is the nucleus (the control room), which houses the cell’s DNA (the house rules or instructions for running the cell). Other organelles include mitochondria (the power generators), lysosomes (the waste disposal units), and ribosomes (the tiny factories where proteins are made). Humans, animals, plants, and fungi are all made of eukaryotic cells.
Prokaryotic Cells (The Studio Apartment): These cells are like studio apartments – simple and open plan. They don’t have any separate rooms or organelles. Everything happens in one space. The DNA is not kept in a nucleus but floats freely in the cell. But despite being much simpler, they can still carry out all the functions necessary for life. Bacteria and archaea are examples of organisms made of prokaryotic cells.
So, eukaryotic cells are like fancy mansions with lots of special rooms for different functions, and prokaryotic cells are like simple studio apartments where everything happens in one place. But whether they’re mansions or studios, each cell type is perfectly adapted to suit the needs of the organism it belongs to.
What is the Role of the Nucleus in a Cell?
Let’s think about the cell as if it were a country. In every country, there’s a government that makes important decisions and gives instructions to its citizens on how to run things. In the world of the cell, the nucleus is like the government.
The nucleus holds the cell’s DNA, which is kind of like the constitution or set of rules for the cell. This DNA holds the instructions for making everything the cell needs. When the cell needs to build something, like a protein, the DNA in the nucleus has the blueprint for it.
These blueprints are sent out from the nucleus to the rest of the cell through tiny molecules called messenger RNA. You can think of this RNA as diplomats or messengers who take the government’s orders and bring them to the citizens – or in this case, to the ribosomes, which are like the factories where proteins are made.
So, the nucleus, in a way, is the cell’s command center. It holds the cell’s genetic material and gives orders to the rest of the cell on how to function and what to build. Without the nucleus, the cell wouldn’t know what to do, and it couldn’t survive. It’s like a country without a government – there would be no rules or structure, and things would quickly descend into chaos.
What is Mitosis?
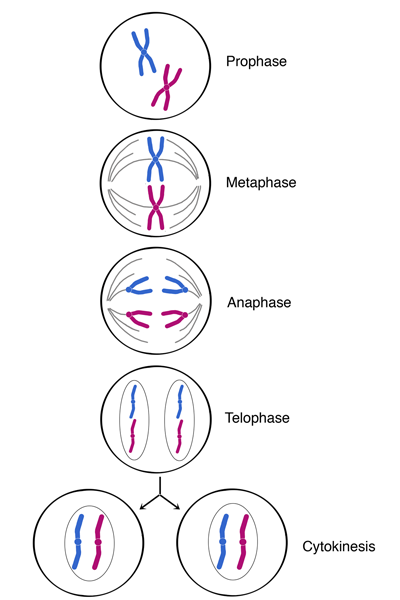
Imagine you’re baking a cake, and the end goal is to have two identical cakes. Sounds impossible, right? But that’s exactly what your cells do all the time through a process called mitosis.
Mitosis is like a cell’s recipe for making a copy of itself. This isn’t any old photocopy, though – it’s a perfect clone, an exact copy of the original. This is crucial for growth and repair in your body. Scratched your knee? No worries, your cells are on it, going through mitosis to patch up the wound with new skin cells. Growing taller? Again, it’s mitosis at work, building more of you as you grow.
The process of mitosis is kind of like a cake recipe with several steps:
1. Prophase (Mixing Ingredients): In the cell, the DNA ‘recipes’ are coiled up tightly into structures called chromosomes. The cell prepares to divide.
2. Metaphase (Lining up in the Baking Tin): The chromosomes line up along the center of the cell. This ensures each new cell will get one copy of each chromosome.
3. Anaphase (Splitting the Cake Batter): The chromosomes are pulled apart to opposite ends of the cell. Now, each half of the cell has a full set of chromosomes.
4. Telophase and Cytokinesis (Baking and Cooling): The cell splits into two, each with its own nucleus. The DNA uncoils and the cell returns to normal, just like a cake cooling and settling after coming out of the oven.
And voila, one cell has become two identical cells. This is mitosis – the recipe of life, that happens millions of times every day in your body. It’s nature’s incredible way of ensuring you grow, develop and heal!
What is the Role of Mitochondria in Cells?
You know that feeling when your phone is at 1% and you’re rushing to find a charger? Now imagine if your cells ran out of power. Not a good situation, right? But don’t worry, your cells have their very own power stations to avoid such a crisis – these are called mitochondria.
Think of mitochondria as the cell’s power generators. They’re like tiny biological power stations, turning the food you eat into fuel that the cell can use. This fuel is called ATP (adenosine triphosphate) and it powers pretty much everything that happens in the cell.
It’s like charging your phone – once it’s charged, you can do all sorts of things with it, from sending texts to browsing the internet. Similarly, once the mitochondria produce ATP, your cell can do all the things it needs to do, from moving and growing to repairing damage and communicating with other cells.
Without mitochondria, our cells wouldn’t have the energy they need to function, and we wouldn’t be able to do… well, anything really! So next time you’re charging your phone, spare a thought for your hard-working mitochondria, tirelessly generating energy to keep you going.
What is the Function of Ribosomes?
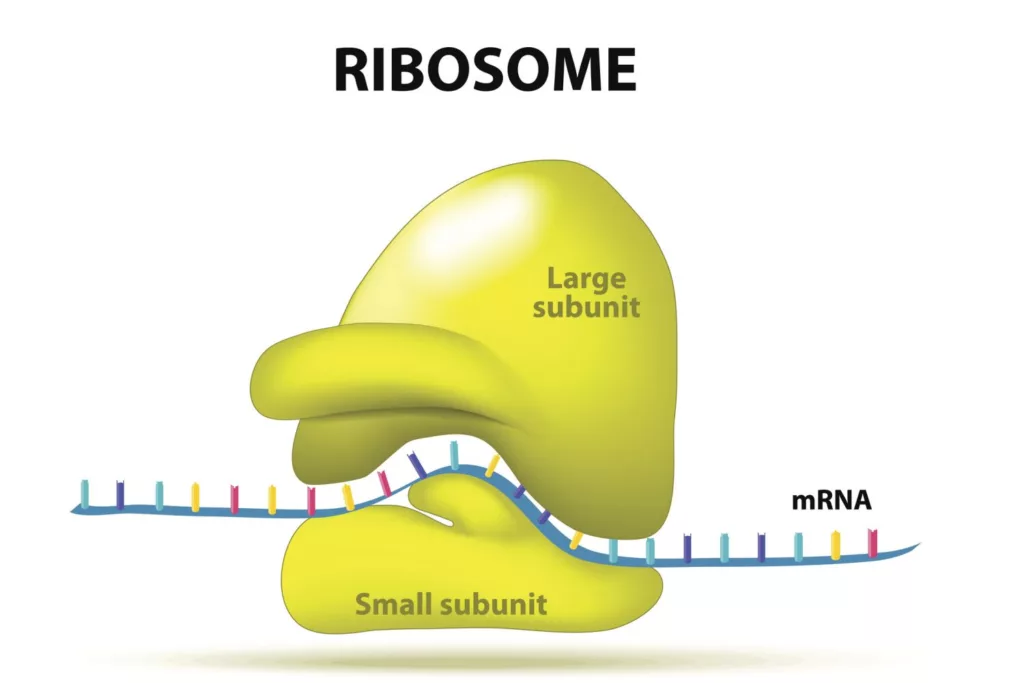
You know when you order something online, and it arrives at your house perfectly made and ready to use? Ribosomes in your cells do something similar – they’re the cell’s assembly line, putting together all the proteins your cell needs.
These proteins aren’t just any old product. They’re like the cell’s tools, machinery, and even building materials, needed for pretty much everything the cell does. Need to send a signal to another cell? There’s a protein for that. Need to speed up a chemical reaction? There’s a protein for that too. Building new parts of the cell? Yep, you guessed it, proteins again!
How do ribosomes know what protein to make? That’s where the cell’s nucleus comes in, sending out instructions in the form of mRNA (messenger RNA). It’s like an online order that comes with a specific list of components and an assembly guide. The ribosomes read these instructions and assemble the proteins accordingly, adding one piece at a time until the protein is complete.
So, ribosomes are like the cell’s manufacturing plant, taking orders from the nucleus, and assembling the proteins that the cell needs to function. Without ribosomes, the cell wouldn’t have the proteins it needs to do its job, which would be like a car factory without an assembly line – not much use at all!
What is Diffusion and Osmosis?
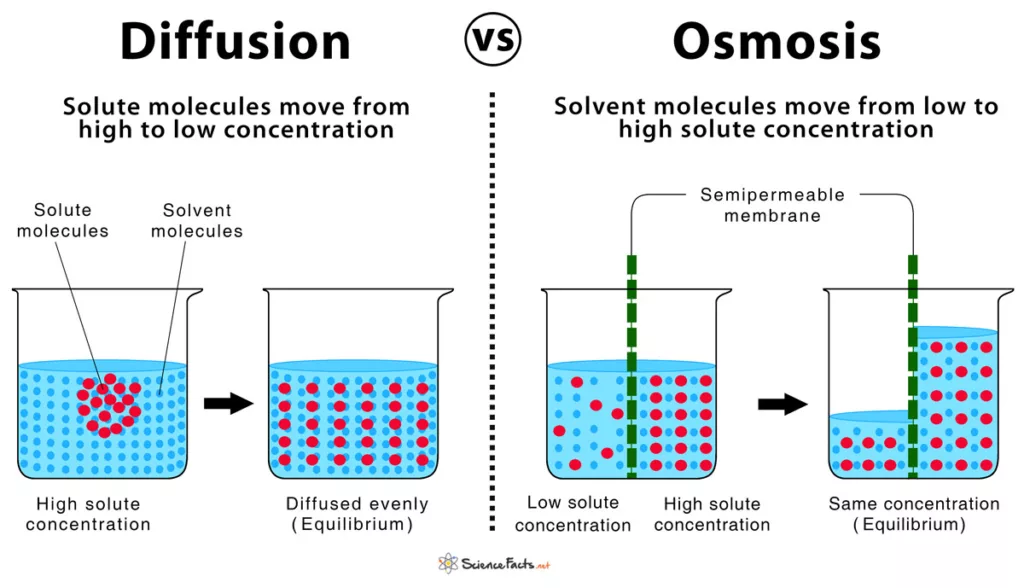
Have you ever sprayed your favourite perfume or cologne in a room and noticed how the scent eventually fills up the entire space? Or maybe you’ve dropped a single drop of food colouring in a glass of water and watched it swirl and spread until the whole glass is coloured. These are everyday examples of a process called diffusion.
Diffusion is like the ultimate sharing system of nature. It’s a process where particles move from an area where they’re packed closely together (high concentration) to an area where they’re spread out (low concentration), until they are equally distributed. It’s as if the particles are saying, “Hey, it’s crowded here, let’s move somewhere we have more space!”
Now, let’s talk about osmosis. Imagine you’re really thirsty and you find a big jug of water. You’d naturally want to drink it, right? Well, in a way, osmosis is how water quenches its thirst. It’s a special type of diffusion where water moves across a semi-permeable membrane (a barrier that only lets certain things through) from an area of low solute concentration (more water) to an area of high solute concentration (less water). It’s as if water is trying to dilute the area where there’s too much solute, to create balance.
Both diffusion and osmosis are all about creating balance, or equilibrium. In our bodies, these processes help to transport nutrients, get rid of waste, and maintain a healthy water balance. Nature is pretty awesome, isn’t it?
What is Active Transport?
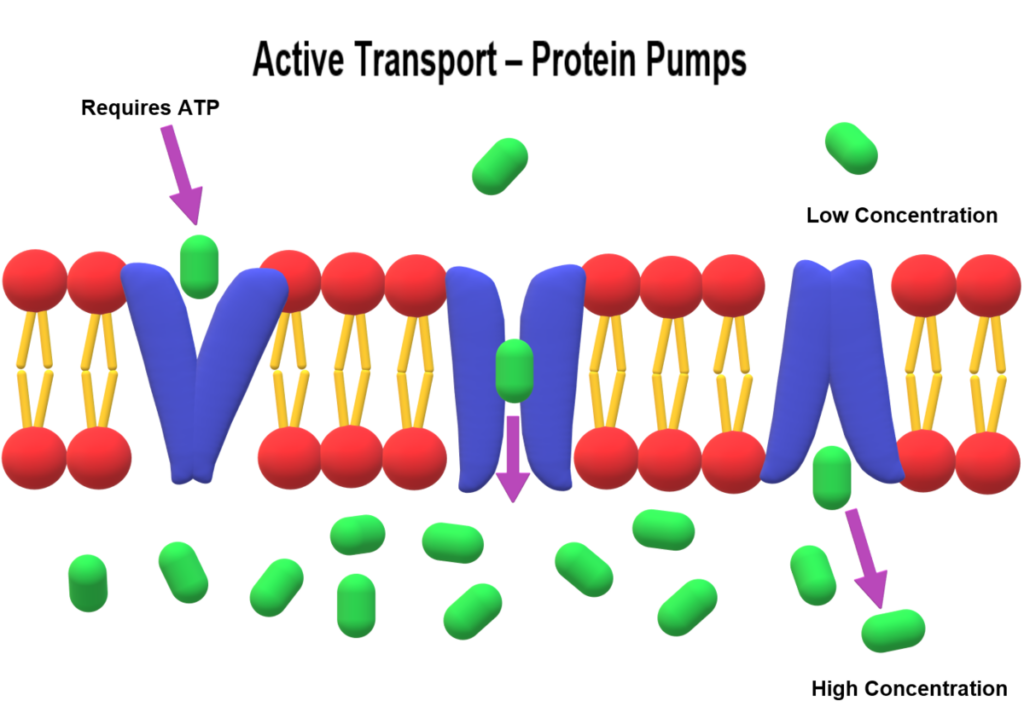
Have you ever tried to push your way through a really crowded place, like a concert or a packed subway train? It takes a lot of energy, doesn’t it? This is kind of what cells have to do when they need to move substances from an area of low concentration to an area of high concentration, a process known as active transport.
Unlike diffusion, where particles naturally move from a crowded area (high concentration) to a less crowded area (low concentration), active transport is like swimming against the current. It’s the cell’s way of saying, “I know it’s crowded there, but I really need to get this substance to that side.”
Here’s the interesting part: this process requires energy. Cells use ATP (adenosine triphosphate), the energy currency they get from mitochondria, to power this movement. It’s like paying a fee to push your way through that crowded subway train.
An example of active transport in your body is when your cells absorb glucose (a type of sugar your body uses for energy) from your blood. Even when there’s more glucose inside the cell than outside, the cell keeps taking in more glucose because it needs a lot of energy.
So, active transport is like the cell’s VIP ticket, letting it push through the crowd and get substances exactly where they need to go, even if it’s the crowded side of the cell. It’s one of the ways your cells make sure they have everything they need to keep you healthy and energised.
What is the Cell Cycle?
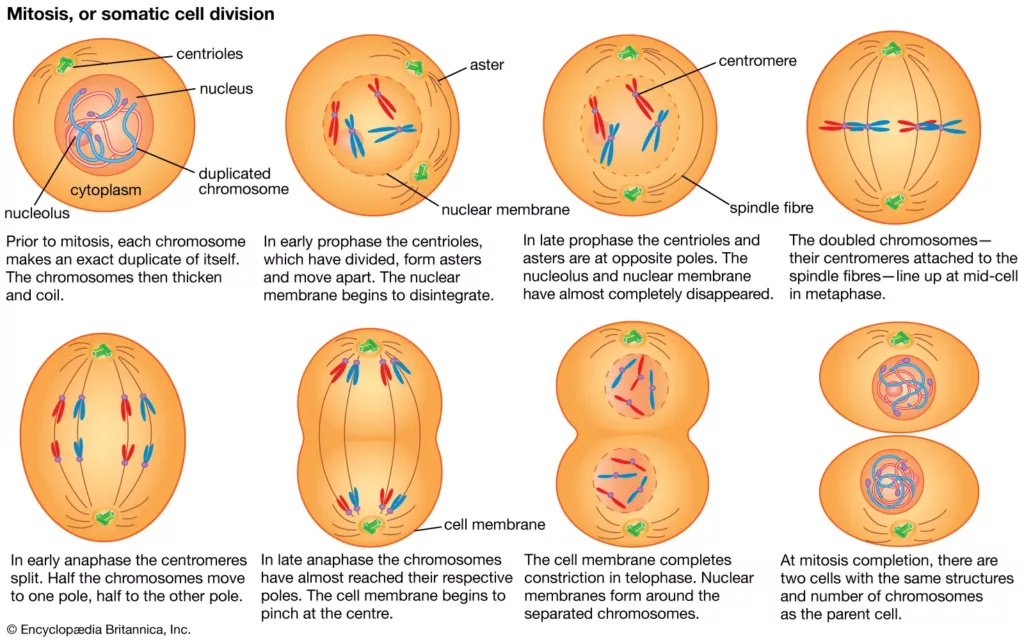
Do you remember when you were little and you kept growing out of your clothes, needing new, bigger ones all the time? Cells in your body go through a similar process of growth and division, called the cell cycle.
Imagine the cell cycle as a journey your cell takes, like a trip around the sun in a year, but instead of seasons, there are different stages.
1. Interphase (Spring and Summer): This is the longest phase, where the cell grows and carries out its regular functions. It’s also preparing for division by replicating its DNA. It’s like a cell’s workday and also preparing for a big trip (the trip being cell division).
2. Mitosis (Autumn): This is when the cell divides its copied DNA and splits in half to create two identical daughter cells. It’s like packing two identical suitcases with everything needed for the trip.
3. Cytokinesis (Winter): This is the final step, where the cell officially splits into two new cells. It’s like finally embarking on the trip, arriving at two new destinations.
These new cells then start their own journeys through the cell cycle, growing, copying their DNA, and eventually dividing to create more cells. This process is key for growth, healing, and sometimes even survival of the organism.
So, the cell cycle is like an epic journey for your cells, where they grow, prepare for division, and then split into new cells, starting the journey all over again!
Test Yourself
If a cell's DNA was damaged, which organelle would be directly affected, and how would this impact the cell?
The nucleus would be directly affected as it houses the cell’s DNA. This could impact cell function as the DNA provides instructions for protein synthesis. Damaged DNA may lead to the production of faulty proteins, which could disrupt various cellular functions.
Why do plant cells have a cell wall, but animal cells do not? What function does this difference serve?
Plant cells have a cell wall to maintain their shape and resist the external pressure, as they need to support the weight of the plant. Animal cells are more flexible and interact with their environment differently, so they don’t require a cell wall.
Describe the path of a protein in a cell from the moment its creation is initiated until it's exported out of the cell.
The creation of a protein starts in the nucleus with the transcription of DNA into mRNA. The mRNA travels to the ribosomes (either free-floating or on the endoplasmic reticulum) where it’s translated into a protein. If the protein is to be exported, it enters the rough ER where it’s modified, then it’s sent to the Golgi apparatus for further modification and packaging into a vesicle. This vesicle merges with the cell membrane, releasing the protein outside the cell.
During a lab experiment, you notice that a cell is not dividing even though it's in an optimal environment for growth. Which phase of the cell cycle might it be stuck in and why?
The cell might be stuck in the G1 phase of the interphase. This is when the cell checks for DNA damage before proceeding to the S phase (DNA replication). If there’s damage, the cell will try to repair it before moving forward, or it might enter a state of dormancy (G0 phase).
Explain the process of active transport using the example of glucose uptake in cells.
Active transport of glucose happens against the concentration gradient, meaning from an area of low concentration to an area of high concentration. This process requires energy (ATP), which changes the shape of the glucose transporter protein embedded in the cell membrane, allowing glucose to be moved into the cell.
Suppose a cell was placed in a solution of sugar water. Over time, the cell shrivels up. Was the solution hypotonic, hypertonic, or isotonic relative to the cell’s cytoplasm? Explain your answer.
The solution was hypertonic to the cell’s cytoplasm. This means it had a higher concentration of solutes (sugar) than the cell’s cytoplasm. Therefore, water moved out of the cell by osmosis, causing the cell to shrivel up.
Why is mitosis crucial for multicellular organisms?
Mitosis is crucial for multicellular organisms for growth (increasing the number of cells), repair (replacing damaged cells), and reproduction (for some organisms that reproduce asexually).
Compare and contrast the structure and function of eukaryotic and prokaryotic cells.
Eukaryotic cells have a nucleus and membrane-bound organelles, while prokaryotic cells don’t. Eukaryotic cells are typically larger and more complex, making up organisms like plants and animals. Prokaryotic cells are smaller and simpler, making up organisms like bacteria.
How do ribosomes know which proteins to produce and when to produce them?
Ribosomes know which proteins to produce and when to produce them based on the mRNA received from the nucleus. When a cell needs a certain protein, the appropriate gene in the DNA is transcribed into mRNA in the nucleus. The mRNA carries this message to the ribosomes, which translate the message and assemble the specified protein.
What would happen to a cell if the mitochondria stopped working properly? How would this affect the organism?
If mitochondria stopped working, the cell would not produce enough ATP (energy), which would affect all cellular activities. In the organism, this could cause energy-demanding processes to slow down or stop, possibly leading to disease.
Additional Knowledge
Cell Theory
The Cell Theory consists of three main points:
- All living organisms are composed of cells: This means that whether we’re talking about a bacteria, a fern, a frog, or a human, all life is fundamentally made up of cells. From the tiniest microorganisms to the biggest mammals, cells are the basic building blocks of life.
- The cell is the basic unit of life: This means that the cell is the smallest entity that can still be considered alive. It’s the simplest thing that can carry out all of life’s processes, like growth, metabolism, and response to stimuli. Think of a cell as the smallest ‘bite’ of life – it contains all the ingredients needed for life, just like a bite of cake contains all the flavors of the whole cake.
- All cells come from pre-existing cells: This means that cells reproduce by dividing into two, with each new cell inheriting a complete set of genetic material from the parent cell. This principle replaced the old belief in spontaneous generation, where it was thought that life could spontaneously arise from non-living matter.
This theory has stood the test of time because it explains such a wide range of observations, and no evidence has been found that contradicts it. The Cell Theory has been expanded and refined over the years, but these three principles remain the cornerstone of biological science.
Structure and function relationship
Structure-Function Relationship: This fundamental principle states that the structure of an object or a living thing often determines its function, or what it can do. Basically, how something is built (structure) affects what it can do (function).
Let’s see a few examples in cell biology:
- Nerve Cells (Neurons): Neurons have long, wire-like structures called axons, which can carry electrical signals over long distances. This structure is perfect for their function of transmitting information around the body.
- Red Blood Cells: These cells are thin and flat to increase their surface area, allowing them to absorb and deliver oxygen more effectively. They also lack many common cell components, like the nucleus, which provides more space for hemoglobin – the protein that binds and carries oxygen.
- White Blood Cells: These cells often have a flexible shape allowing them to squeeze through narrow blood vessels and reach the site of infection, aligning with their function of fighting diseases.
- Epithelial Cells: These cells in your skin, intestines, and other areas are tightly packed together to form a barrier against physical damage, pathogens, and water loss.
In the world of proteins (the workhorses inside cells), the structure-function relationship is especially crucial. The shape of a protein – its 3D structure – is intimately tied to what it can do. For example, enzymes are proteins that catalyze (speed up) chemical reactions. Their function depends on their structure, which allows them to bind specific molecules and convert them into other molecules.
So, in essence, the structure-function relationship is a fundamental principle in biology that explains how the architecture of a cell or its components enables it to perform its specific tasks. It’s a little like looking at a tool – once you know what a hammer or a pair of scissors does, you can understand why it’s shaped the way it is.
Specialisation (Differentiation) of Cells
This is the process where generic cells develop into cells with specific functions, a bit like choosing a career! In a multi-cellular organism like a human, there are many types of cells, each with a specific role to play in keeping the organism alive and well.
Think of your body as a big, bustling city. Just as a city needs different professionals – like firefighters, teachers, doctors, and chefs – to keep it running smoothly, your body needs different types of cells.
Here are some examples:
- Red Blood Cells: These cells are specialists in transport. They have a unique, disk-like shape and are filled with a molecule called hemoglobin that can carry oxygen from your lungs to the rest of your body.
- Nerve Cells (Neurons): These are like the electrical engineers of your body. They have long, thin structures that can carry electrical signals from one part of your body to another, helping you react to your environment.
- Muscle Cells: These are the movers and shakers! They are elongated and filled with proteins that can contract, allowing you to move your body.
- White Blood Cells: These cells are your body’s security team. They can engulf harmful bacteria or produce antibodies to fight viruses.
- Sperm and Egg Cells: These are your body’s reproductive specialists. They carry half of your DNA and can combine to create a new organism.
But here’s the cool part – all these specialized cells originally come from the same type of cell: a stem cell. Stem cells are like kids who haven’t chosen a career yet. Under the right conditions, they can differentiate into any type of cell, whether it’s a neuron, a muscle cell, or a red blood cell. This process is controlled by the activation and deactivation of different genes within the stem cell.
So, cell specialization or differentiation is the process where cells develop specific structures and abilities, taking on various roles to help your body function as a whole. Just like a diverse team of professionals in a city, a variety of specialized cells is crucial to keep your ‘body city’ running smoothly!
Homeostasis
Homeostasis: This term describes the process by which living organisms maintain a stable internal environment, even when their external environment changes. In essence, it’s all about keeping things constant and balanced. The term comes from the Greek words for “same” and “steady.”
Let’s think about your own body as an example. You’re a living organism, so you need homeostasis to survive. Here are some examples:
- Body Temperature: Humans need to maintain a body temperature around 37°C (98.6°F). If you get too hot, you start to sweat, which cools you down. If you get too cold, you start to shiver, which warms you up. This is homeostasis in action.
- Blood Sugar Levels: Your body needs to keep the amount of sugar in your blood (glucose) balanced. If you eat a meal, your blood sugar levels rise. Your body responds by releasing insulin, which allows your cells to take in glucose and bring your blood sugar levels back down.
- Water Balance: Your body also has to maintain the right balance of water. If you’re dehydrated, your body signals thirst, making you want to drink. If you’ve drunk too much, your body produces more urine to get rid of the excess water.
These are just a few examples, but virtually every part of your body is involved in homeostasis in one way or another.
In cells, homeostasis involves maintaining the balance of ions, nutrients, and other substances within the cell and in the fluid surrounding the cells. This allows cells to function optimally despite changes in their environment.
Failure of homeostasis can lead to disease or even death. For example, diabetes is a disease caused by the failure of blood sugar homeostasis.
So, you can think of homeostasis as the “Goldilocks Principle” for living things – keeping everything not too high, not too low, but just right.
DNA Replication
DNA Replication: This is the process by which a cell makes a copy of its DNA. Each of your cells (with some exceptions like red blood cells) contains DNA, a long molecule that holds the instructions, or “blueprint,” for building and operating your body. DNA replication is how cells copy these instructions so they can be passed on to new cells during cell division.
Picture DNA as a ladder twisted into a spiral, known as a double helix. The sides of the ladder are made up of sugar and phosphate molecules, and the rungs are made up of pairs of four different molecules called bases (adenine, thymine, cytosine, and guanine), which pair in a specific way: adenine with thymine, and cytosine with guanine.
Here’s a simplified rundown of how DNA replication works:
- Unzipping: The first step in DNA replication is unwinding the double helix. An enzyme called helicase breaks the hydrogen bonds between the base pairs, causing the two strands to separate, like unzipping a zipper. This forms a ‘replication fork,’ a Y-shaped structure where new DNA is synthesized.
- Building new strands: Each of the two separated strands serves as a template for building a new strand. Enzymes called DNA polymerases move along the strands, ‘reading’ the sequence of bases and bringing in new bases to form base pairs with the template strand. Because of the specific base pairing rules (A with T, and G with C), the sequence of each original strand is preserved in the new strand.
- Zipping up: Two identical DNA molecules are formed, each composed of one original and one new strand. These molecules then wind up again into the familiar double helix structure.
This process is remarkably accurate, but occasional mistakes, or mutations, can occur. Most of these are repaired by the cell’s machinery, but some can persist, and over time, these can lead to evolutionary changes.
So, DNA replication ensures that every new cell has an exact copy of the DNA from the parent cell. It’s like a molecular photocopier, duplicating the DNA blueprint every time a cell divides.
Gene Expression
Gene Expression: Gene expression is the process by which the information stored in a gene is used to create a functional product, typically a protein. The DNA sequence of a gene is first transcribed into RNA, which is then translated into a protein. This two-step process is often summed up as “DNA makes RNA makes protein.”
Here’s a bit more detail on how this works:
- Transcription: During transcription, an enzyme called RNA polymerase attaches to the DNA at the start of the gene. The RNA polymerase “reads” the DNA sequence and uses it as a template to create a strand of messenger RNA (mRNA), a molecule similar to DNA but with some chemical differences. The mRNA carries a copy of the gene’s information.
- Translation: After transcription, the mRNA leaves the nucleus (where the DNA stays) and travels to the ribosomes, which are the cell’s protein-making factories. At the ribosome, the mRNA sequence is translated into a sequence of amino acids, which are the building blocks of proteins. Each set of three bases in the mRNA, known as a codon, corresponds to a specific amino acid.
The sequence of amino acids determines the structure and function of the protein. Once the protein is produced, it can go on to carry out its job in the cell, whether that’s helping to break down food, protect the body from infections, or any number of other functions.
It’s important to note that not all genes are expressed (used to make protein) at all times. Cells control when and where genes are expressed, so the right proteins are made in the right places at the right times. For example, the genes expressed in a heart cell are different from those expressed in a skin cell or a liver cell, even though the DNA in these cells is identical.
So, gene expression is like the cell’s recipe for making proteins. The DNA holds the instructions, the mRNA carries those instructions to the ribosomes, and the ribosomes use those instructions to build the protein. This process is critical for the cell to function correctly and for the organism to survive and reproduce.
Apoptosis
Apoptosis: This is a form of programmed cell death – think of it as a cell’s way of committing suicide. But it’s not as grim as it sounds! Apoptosis is a normal and essential process for the health and development of all multicellular organisms, from humans to plants.
Here’s why apoptosis is so important:
- Development: Apoptosis helps shape our bodies as we grow. For example, when human embryos are developing, their fingers and toes are initially webbed. Apoptosis kills the cells in the webbing, and voila, you have separate fingers and toes!
- Cell turnover: Our bodies are continually producing new cells, so old or damaged ones need to be removed to make space for the new ones. Apoptosis is the clean and tidy way to do this. For example, red blood cells are replaced approximately every 120 days through this process.
- Defense against disease: If a cell becomes infected by a virus or undergoes changes that could lead it to become cancerous, apoptosis can eliminate the threat by killing the problematic cell.
How does apoptosis work? It’s triggered by signals within the cell or from the cell’s environment. These signals activate a series of proteins within the cell, which leads to the cell’s death. Importantly, the dying cell sends out signals to immune cells, which come and engulf the cell debris, cleaning up after the cell’s demise.
So, while apoptosis might sound a bit morbid, it’s a vital process for keeping organisms healthy. It’s a bit like taking out the trash – by getting rid of cells that are no longer needed or could cause trouble, apoptosis helps keep the body in balance.
Stem Cells
Stem cells are like the wild cards of the cellular world. They have two key properties that set them apart from other cells:
- Self-Renewal: Stem cells can divide and produce more stem cells. They can replicate many times, a property called proliferation.
- Differentiation: Stem cells can also transform, or ‘differentiate’, into specialized cells with specific functions, like heart cells, skin cells, or neurons. They’re a bit like a box of lego blocks that can be built into a spaceship, a dinosaur, or a castle.
Based on their potency, or their ability to differentiate into different cell types, stem cells can be classified as:
- Totipotent: These stem cells can grow into a whole new organism! The fertilized egg and the cells produced by the first few divisions of embryonic development are totipotent.
- Pluripotent: These stem cells can turn into almost any type of cell. Cells of the early embryo (called the blastocyst) are pluripotent.
- Multipotent: These stem cells can become a select few types of cells. For example, blood stem cells, which can create red blood cells, white blood cells, and platelets, but not other types of cells.
- Unipotent: These can only produce cells of their own type, but they do self-renew and are therefore still considered stem cells.
Stem cells are crucial for growth and development, and they also help repair tissues in the body. In addition, scientists are studying stem cells to understand diseases, develop new treatments, and potentially even grow new organs for transplants. Despite their enormous potential, the use of stem cells also raises ethical issues, particularly when it comes to embryonic stem cells.
In a nutshell, stem cells are the foundation upon which the body is built, and their unique properties make them a powerful tool in biology and medicine.
Surface Area to Volume Ratio
Surface Area to Volume Ratio (SA:V): In the simplest terms, this refers to the relationship between the surface area of an object (the amount of space on its outside) and its volume (the amount of space it takes up).
As the size of an object increases, its volume grows much faster than its surface area. This is because volume is related to the cube of the size (length x width x height), whereas surface area is related to the square of the size (length x width).
This concept has important implications in biology, particularly for cells:
- Nutrient Absorption and Waste Removal: Cells take in nutrients and expel waste products through their surface. Therefore, a cell with a larger surface area can absorb more nutrients and expel waste more efficiently.
- Heat Regulation: Cells also regulate heat through their surface. If a cell’s volume is too large compared to its surface area, it can’t release heat fast enough and could overheat.
Because of these factors, cells tend to be small. The smaller a cell is, the larger its surface area to volume ratio, and the more efficiently it can function. This is why, for example, the cells lining our intestines have tiny, finger-like projections. These projections increase the surface area of the cells, allowing them to absorb nutrients more efficiently.
So, in a nutshell, the surface area to volume ratio is a key factor in determining the size and shape of cells, as well as their ability to function efficiently. Understanding this concept can help us understand why cells are the way they are and how they interact with their environment.
Energy Conversion
This refers to the process of changing energy from one form to another. Energy exists in several forms, including kinetic, potential, thermal, gravitational, sound, light, elastic, and chemical energy. The Law of Conservation of Energy states that energy cannot be created or destroyed; it can only be transferred or converted from one form to another.
In the context of biology, energy conversion is a fundamental process that sustains life. Here’s an example:
Photosynthesis: This is the process used by plants, algae, and certain bacteria to convert light energy, usually from the sun, into chemical energy in the form of glucose (a type of sugar). The organisms can then use this glucose for their metabolic processes, or “life functions.” In a way, these organisms are like little solar power plants, capturing and converting sunlight into usable energy!
Here’s another important example:
Cellular Respiration: This process takes place in the cells of organisms, including humans. It involves converting the chemical energy in glucose and other nutrients into a form of energy called adenosine triphosphate (ATP), which cells can use to power their activities. It’s kind of like a power plant burning coal or gas to produce electricity.
In both photosynthesis and cellular respiration, not all energy is converted to a useful form; some is lost as heat, which is a less usable form of energy. This concept is illustrated by the second law of thermodynamics, which states that energy conversions are never 100% efficient and that some energy is always lost as heat.
So, energy conversion is a critical process in biology that allows organisms to harness energy from their environment and use it to sustain life. From sunlight to sugars to cellular fuel, life is all about transforming energy!
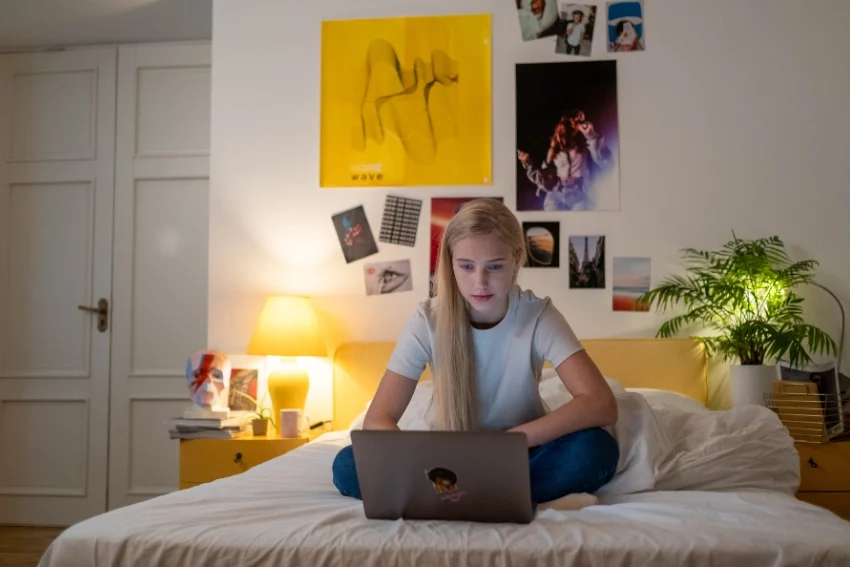